The dirt on our soils
Expert reviewers
Essentials
- Soils are a precious resource—we depend upon soils, so they need to be used sustainably and the salts within them managed
- Salinity is a problem that is not restricted to agricultural regions—wetlands, river systems and urban environments can all be affected
- Dryland salinity occurs when landclearing or irrigation result in the water table rising closer to the surface, bringing salt with it
- Transient salinity occurs when salt accumulates in the root zone of plants during dry periods
- Sodicity occurs where too much sodium in the soil fundamentally changes the soil structure
- A wet sodic soil will be slippery but prevents water infiltrating to the deeper soil layers, while dry sodic soils can form crusts as hard as concrete
- Sodic soils result in increased runoff and severe erosion
With an area of around 7.5 million square kilometres, mainland Australia has a lot of soil. But around 70 per cent of our continent is classified as arid or semi-arid, so there’s not all that much productive soil available for the agriculture we depend on. The soil we do have is precious, and farmers can face significant challenges maintaining its quality.
Australia’s soils are old. During the last ice age (around 20,000–26,000 years ago) many land masses were covered by ice sheets, which scraped away the soil as they moved over the continents. As the exposed fresh rocks weathered, new and nutrient rich soils were created.
This was not the case here in Australia. Not much of our continent was covered by ice during the last ice age, and so our soils have been sitting around for a very long time, slowly undergoing more and more weathering and erosion. This weathering has dissolved vast quantities of nutrients, and washed them away into the groundwater and/or rivers, leaving the soils depleted in nutrients such as phosphorous, calcium and potassium. The productivity of our old, nutrient-poor soils has also suffered because of some land management practices introduced since European settlement.
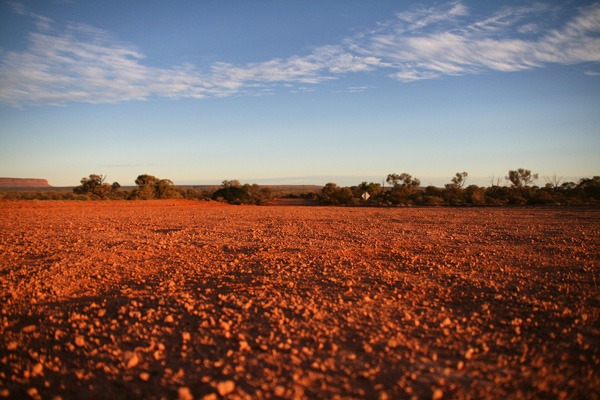
Salinity
When we think of salt, we generally think of sodium chloride—NaCl, common table salt—and this is the most common form of salt that affects our soils. However, it’s not the only one, although most are salts of sodium (Na+). Also present are sodium carbonate (Na2CO3) and sodium bicarbonate (NaHCO3).
A soil or water which has high concentrations of salt is described as saline. Salinity occurs naturally in much of Australia’s landscape—think of the great salt lakes such as Lake Eyre in our interior. Many of our agricultural lands also contain vast reservoirs of salt, although these are usually held deep within the soil profile, below the root zone, so they don’t affect plant growth. The salt is often found within aquifers (porous rocks capable of storing water).
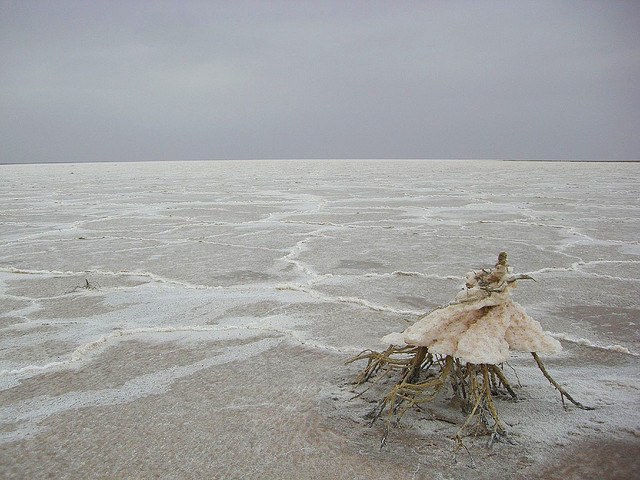
The salt found in the landscape has come from several sources. In Western Australia, the main source is the ocean—salt is carried inland by the wind, and deposited on the land by rainfall and dust. Salt that arrives in this way is called cyclic salt. Over millions of years, large amounts of cyclic salts have been deposited across Australia.
Some salt in the soil profile may date back even further, to when the parent rocks themselves were formed. These rocks release salts as they weather away. Other sources of salt are ancient drainage basins or inland seas that evaporated water over long periods of time when Australia was more arid, forming salt deposits which still remain today.
Groundwater systems
To understand how salinity forms in our soils, we first need to understand how water, which carries salt with it, moves through the soil profile.
When water moves from a river or from above the ground into the soil and rocks below, it usually travels through or fills up the tiny spaces (pores) between soil particles. When these pores are filled with water (saturated) the water is called groundwater. The top of the saturated zone is called the watertable.
A groundwater body is water contained within an aquifer—rocks which are saturated with water so that a pump can pull water from them. The water which occurs in aquifers is termed a groundwater body. These groundwater bodies are recharged whenever water infiltrates deep enough to reach them or sometimes when people pump water into them. If groundwater leaves the aquifer—perhaps when it flows into a river system, or is forced to the surface as springs—this is called discharge.
Groundwater systems can vary greatly in size and dynamics. Local groundwater bodies usually occur in the hilly terrain in the upper parts of a catchment and on a horizontal scale of about one to three kilometres. Because of their relatively small size and the poor connection between the pores in the soil and rocks (i.e. low permeability of the underlying rocks), these systems rapidly ‘fill up’ with water after land-clearing, they respond relatively rapidly to changes in recharge and discharge.
Intermediate groundwater systems occur in the mid and lower areas of large catchments on a horizontal scale of 10 to 15 kilometres. The groundwater in these systems is sourced from infiltration and from the local systems which occur uphill.
Regional groundwater systems occur in flat terrain, and can overlap multiple catchments as they occur beneath mountain ranges and hills. They usually occur on a scale of 50 kilometres or more.
Groundwater dynamics in regional groundwater bodies can be very slow. The terrain in regional systems is often quite flat, so the flow of groundwater to low points in the catchment and subsequent drainage out of the system can be very slow. The time between water entering a regional system and later leaving as discharge can be tens of thousands of years.
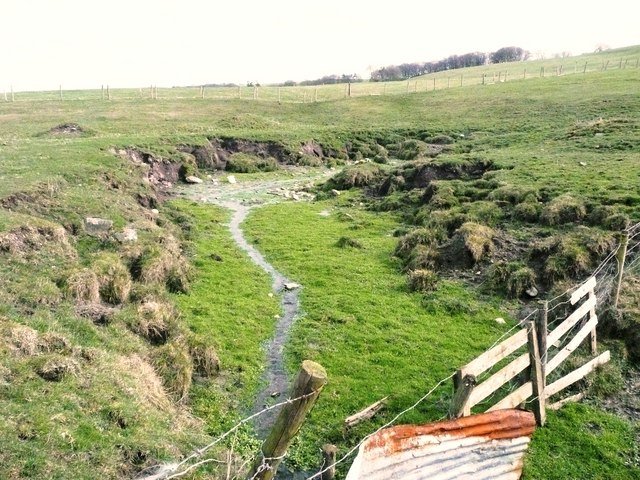
Dryland or seepage salinity
Land clearing, irrigation or extreme rainfall cause more water to be added to both rivers and the groundwater systems. This makes the watertable rise closer to the surface. A rising watertable can dissolve salt present in the soil profile and carry it to the surface. This is known as dryland or seepage salinity.
The salt will cause problems as it enters the root zones of the plants, or reaches the surface. In the past, the deep roots of native woodlands and forests used up most of the water entering the soil profile, keeping both the watertable and the salt sitting deep in the soil profile stable. The salt stayed quite harmless where it was.
When Europeans arrived and began clearing native vegetation and planting more shallow-rooted plants such as agricultural crops, this changed. The annual crops and pastures that replaced the native vegetation used less water as they only grew for part of the year and their shallow roots did not absorb water from very deep below the soil surface. Groundwater recharge increased and caused the watertable to rise. As it rose, it carried the dissolved salt which was previously lying harmlessly in the soil profile. This caused salinity to increase as the groundwater evaporated and salt was left behind.
Salt affects the growth of plants and sometimes can crystallise on the surface, leaving an area where nothing will grow. This is called a scald.
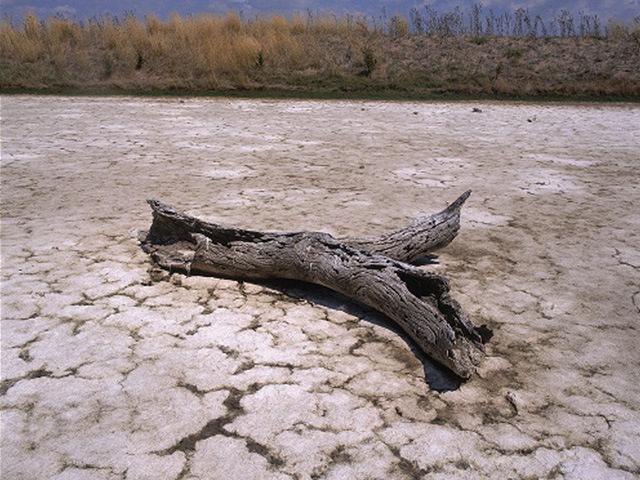
Irrigation salinity is caused by a similar effect—the application of excess water to irrigate crops causes the watertable to rise. The problem is made worse if the irrigation water itself is also saline. Although irrigation in some areas of the Murray Darling Basin in New South Wales has resulted in around 4800 hectares of agricultural land being affected by irrigation salinity, much of Australia’s agriculture is under dryland conditions, where no irrigation occurs and the only water received is rainfall. Irrigation salinity is therefore not so much of a problem in Australia.
Transient salinity
An even more widespread, but less obvious salinity problem is caused by salt that accumulates in the plant root zone without the influence of a rising watertable. Known as transient salinity, or subsoil salinity, it is common in soils in areas of low rainfall, and affects nearly 70% of Australia’s dry-land cropping regions, costing millions of dollars a year in decreased agricultural yields. The lack of rainfall in these areas means that salts don’t get flushed through the soil profile effectively, and instead accumulate in the plant root zone. Transient salinity often occurs where the watertable is deep below the ground surface and an impermeable layer of soil prevents salt leaching through the soil profile even when there is sufficient water present. The lack of leaching further contributes to salt accumulation.
Salt accumulates
Although the concentration of old, cyclic salts in rainwater and most surface waters is very low—only a few parts per million, which is completely harmless to plants. However, when plants take water from the soil profile, the salt gets left behind. Over millenia, the concentration of the salts in the soil builds up.
A salty drink
For plants
Plants don’t like salty water for a few reasons. The biggest problem is that salt molecules are hydrophilic—they attract water molecules. A salty soil retains the water molecules that the plant roots are trying to suck up. In such salty soils, the plant will have to expend more energy to get the water. This affects plant growth, sometimes to the extent that plants die. It’s been estimated that salinity can cost many millions of dollars a year in decreased crop yields.
The other reason is that although most plant roots are very effective at excluding salt from the water they absorb, eventually they will be unable to keep out the sodium ions. When too many of these sodium ions are taken up they become directly toxic to the plant.
The ability to exclude salt varies considerably between different plant species, and scientists are working on breeding new strains of wheat that are more efficient at excluding salt and can better tolerate saline soils. Some plants, like saltbush (Atriplex sp.) or mangroves (Avicennia sp.) are extremely good at coping with saline conditions. They are able to exclude salt not only from entering their roots, but they can extrude excess salt through their leaves, where it forms small crystals.
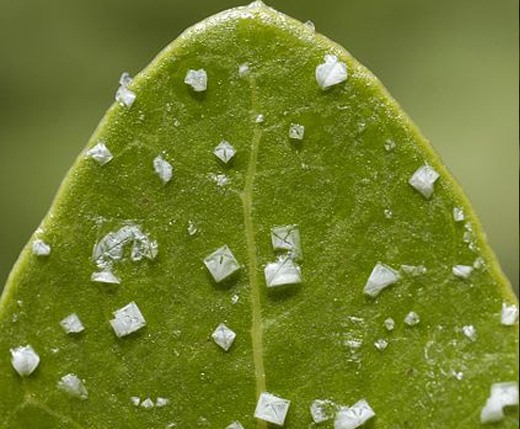
For us
People don’t really like drinking salty water either. According to the Australian Drinking Water Guidelines written in 2011, drinking water should have less than 0.6 grams of solute in every litre, or 600 parts per million (ppm). These solutes are a combination of sodium, potassium, calcium, magnesium, chloride, sulphate, bicarbonate, carbonate, nitrate, and some organic matter. This value is based upon the taste of the water that most people find palatable, as well as a potential health risk for people with high blood pressure (our kidneys work hard to maintain the correct sodium concentration in our body's fluids, which would otherwise cause high blood pressure, leading to a risk of heart attack). Most Australian cities have drinking water with solute concentrations of between 0.1 and 0.75 grams per litre, while in regional areas solute concentrations may reach up to 1 gram per litre or higher.
The city of Adelaide draws 40 per cent of its drinking water from the Murray River, and up to 90 per cent in times of low rainfall. Large-scale salinisation of the Murray River system would pose problems for the quality of the water supply of Adelaide, so a desalination plant has been installed.
Changes to the ionic balance
The salts in saline soils can sometimes change the ratios of the different dissolved ions in water available to plants. This can affect plants’ ability to absorb the nutrients they need to grow. For example, high levels of calcium can affect the plants uptake of iron (Fe), a problem known as lime-induced chlorosis. A high pH due to excessive bicarbonate (HCO3-) (known as alkalinity) can reduce the uptake of many nutrients. High levels of sodium can result in plants being unable to take up potassium (K),and high levels of chloride can reduce nitrate uptake.
Threat to native species
The environmental impacts of salinity are not confined to agriculture. The biodiversity that remains in the Murray-Darling Basin and the West Australian wheatbelt, which are largely cleared of their original vegetation, is also threatened by saline conditions.
Saline river systems can bind to the clay particles in the water to such an extent that they flocculate—they clump together and settle out of the water. This flocculation means more light can penetrate through the water. This, combined with the increased nutrients caused by agricultural runoff, can lead to algal blooms within the waterways.
Wetland systems can also be vulnerable to salinity, as they are generally located in low-lying areas that receive a lot of drained water. Once they become saline, it can be difficult to effectively flush salt out of the wetland.
Salt in the city
Salinity can also pose problems away from the pasture or the bush, in built environments. Disruption to the water table from development and altered run off regimes can result in what’s known as urban salinity.
The salt found in urban environments generally comes from natural sources, but is added to by things like soaps, detergents, swimming pool chemicals, industrial and domestic waste and sewage. There is a lot of sodium in laundry detergents, which hospitals and hotels/motels use a lot of.
Salt can damage infrastructure, cause increased rusting, corrode concrete and brickworks of buildings. Salty deposits can form on buildings and other structures, and as the salt crystals grow and expand, they can cause damage to bricks, mortar and pipe works. A common problem in saline areas is extensive potholes in road surfaces.
Managing saline lands
Land managers have developed a number of ways to reduce salinity. The best method to reduce salinity is different in each area, depending on how the salinity occurs. Examples of common methods include:
- Reducing the height of water tables by:
- planting perennial, deep-rooted crops such as lucerne and perennial grasses
- better management of annual crops and pastures
- installing systems that drain excess surface and sub-surface water and pump out groundwater
- using living with salt strategies such as planting salt-tolerant crops and grasses
- developing new industries that use the saline resources (such as saline aquaculture and harvesting salt on a commercial basis)
A popular idea was to conduct widespread tree planting in salt-affected areas, but ultimately this plan was largely unsuccessful. The trees were often planted on around salt scalds and died, and other trees planted on tableland areas often simply reduced the flow of fresh water through the system.
These days, dryland salinity is no longer dramatic problem—the fears of the 'great white death' have largely been dispelled, through a combination of solid science and improved agricultural and land management practices.
Sodicity
Salinity's unpleasant cousin
Often coming hand-in-hand with transient salinity in a soil is the problem of soil sodicity, where high sodium concentrations have accumulated in the soil profile. Sodicity affects nearly a third of all soils in Australia, including a third of all agricultural soils, where it can cost as much as $2 billion a year in lost production.
Although sodicity is caused by a chemical problem—too much sodium—its effects are largely physical. Sodicity changes the structure of the soil by preventing the soil particles from forming clumps that allow the water to flow between cracks and pores. Instead, particles in a sodic soil disperse and form sheets into which water cannot penetrate. In wet conditions, sodic soils can be slippery on top and dry underneath, as water can’t infiltrate deeper into the soil profile. In dry conditions, these slippery soils can dry as hard as concrete.
Sodic soils lead to increased erosion and runoff. As rainwater can’t penetrate the soil, it runs along the top, gaining speed as it goes. The faster water erodes deeper and deeper into the soil, creating furrows called rills and gullies, and causing the water flow to speed up even more. In other situations where only the subsoil is sodic, subsurface water flowing over this sodic layer will create tunnels, leaving cavities that eventually collapse to form gullies.
The runoff water often carries fine clay particles into waterways and reservoirs. This is called entrainment, and causes turbidity or cloudiness within waterways. The removal of turbidity is very costly for industrial and domestic water users. It also causes environmental problems in rivers and wetlands as it prevents light from reaching plants that need it and impedes some animals’ ability to hunt for their food. In addition, run-off from sodic soils is more likely to carry higher levels of nitrogen and phosphate into waterways and reservoirs, which can contribute to algal blooms.
If sodicity occurs below the root zones of plants, its effect on crop productivity may be less apparent but it can still cause significant problems. For example, in a high rainfall area on sloping land, subsurface water will be unable to percolate deeper into the soil profile. It can flow over the sodic layer within the soil and be lost in what is termed lateral drainage. On flatter land, the sodic layer may not permit water to drain at all, leading to waterlogging at the surface. In arid and semi-arid regions, the wind can speed up along the soil surface in much the same way that water does. This wind erosion from sodic soils can lead to dust storms, which create major environmental and human problems.
Why sodium causes so much trouble
Clay particles (any particle less than 2 micrometres (0.002 millimetres) is termed a clay) within a soil are made up of many different elements and have an overall negative charge. The build-up of positively charged sodium ions (Na+) is a problem because they are strongly attracted to the negatively-charged clay particles. The clay particles become surrounded by Na+ particles, and this changes the way the clay particles then interact with each other, and the structure of the soil as a whole. The result is that the clay particles lose their tendency to stick together when wet—leading to the unstable soils that erode or become impermeable to both water and roots.
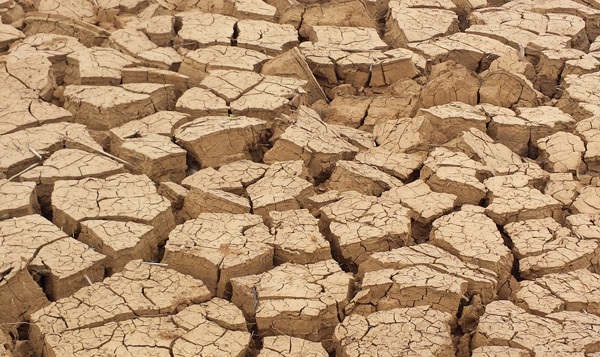
Salinity can suppress sodicity
Sodic soils which are also saline can contain high concentrations of both sodium and sodium chloride. Strangely enough, such soils will usually not exhibit symptoms of sodicity. The presence of both sodium and chloride ions together in the soil solution, rather than a large concentration of just sodium ions attached to the clay particles means the osmotic potential gradient is weakened, less water is drawn in between the clay particles and the soil particles do not become dispersed.
The amount of dissolved ions required to prevent decline in soil structure is called the threshold concentration. It can be calculated quite easily and has been used in the reclamation of soils which have become sodic. The adverse symptoms of sodicity will start to appear if the solute concentration falls below the threshold concentration.
Treating sodicity
Sodicity can often be treated. Most commonly, calcium-containing substances like gypsum (CaSO4) are applied to the affected soil. When gypsum dissolves, it produces calcium ions which then displace the problematic sodium.
Powdered gypsum can be applied to the topsoil, so that the exchangeable sodium in the top 5 centimetres of soil is largely replaced. This may require additions of gypsum of the magnitude of 5 tonnes per hectare.
Another method is known as the electrolyte effect, where calcium is added to irrigation water and again, the calcium displaces the sodium in the sodic soil. This strategy is used where the addition of large quantities of gypsum is uneconomical.
Such additives may not always solve the problem in the long term. For example, very large quantities of gypsum may be needed if the additions are to have anything more than a short-term effect. And sub-soil sodicity may not be affected by the addition of gypsum at the surface, unless the soils are also deep-ripped to aid penetration. Gypsum itself can cause its own problems as it too, is a form of salt.
Large areas of the cropping lands of southeastern Australia have either sodic topsoils or sodic subsoils or a combination of both. Many land managers are using about 5 tonnes per hectare of gypsum every 10 years or so to ameliorate the sodic conditions. Although spectacular improvements have occurred in soil structure and subsequent crop and pasture growth, this is an extremely expensive option and not always economically viable.
As well as improving agricultural profitability, reducing erosion and improving water quality, the application of gypsum has widened land use options and crop types. It has also reduced damage to infrastructure such as roads and buildings.
A combination of solid research and improved land management practices has resulted in the ability of farmers and scientists to keep the challenges posed by salinity and sodicity well in hand. However, our soils are a precious resource, and awareness and vigilance is required to maintain our continent’s arable soils.
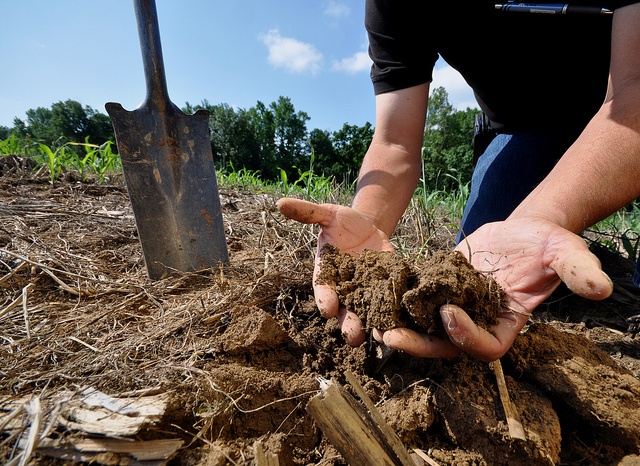