Keeping track of time
Expert reviewers
Essentials
- Different cultures around the world have developed systems to mark the passage of time and record significant events.
- Many of these systems are based on astronomical observations of the sun, moon and stars.
- Other calendar systems, such as those of many Indigenous Australian communities, are based on climatic and ecological observations.
We’re creatures of habit, routine and organisation. We like to know the date of famous events in history, and how long we have to wait until our next birthday party. We arrange with friends to do something ‘next week’, make a dentist appointment ‘for 21 October’ or talk about what we’ll do during our holiday ‘next year’. We take calendars, and the way we use them to keep track of our lives, for granted, but where did these allotments of time that are such a crucial part of how our society functions actually come from?
Lunar vs. solar vs. … lunisolar
Lunar
The changing appearance of the moon in the night sky is one obvious marker of the passage of time. At the start of its cycle (‘new moon’) the moon lies directly between the sun and Earth and we can’t see its illuminated face. As the moon moves in its orbit, we see a crescent. The crescent grows over a period of nights until the entire face can be seen—when it becomes a ‘full moon’. The face then wanes until, once again, it can’t be seen from Earth. This cycle is called a ‘lunation’ and it formed the basic unit (month = moon-th!) of many early calendars. A lunation takes an average of 29.53 days.
The diagram below shows the progression of the lunar phases as seen from the southern hemisphere.
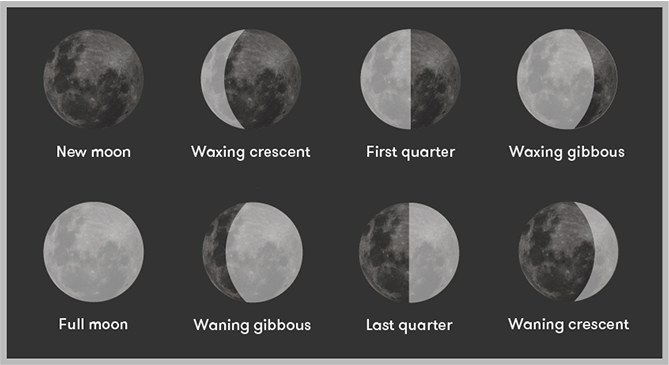
Lunar calendars are problematic, due partly to the fact that the average lunation is not a whole number. If ‘29’ were the number used to mark the lunar month, the calendar would very quickly get out of sync with the actual phases of the moon. The first month would be out of sync by around half a day and the next month by a full day.
Trying to solve the problem by alternating the length of the month between 29 and 30 days, giving an average month of 29.5 days, still results in a calendar that gets out of step pretty quickly, since the actual length of a lunation is a bit more than 29.5. So what happens is that these calendars must be ‘adjusted’ from time to time. This is usually done by periodically adding days (intercalations) or subtracting days (extracalations).
Solar
Other calendars measure time in terms of how long it takes Earth to complete one circle of the sun—this is a solar calendar. Solar calendars have similar issues to lunar calendars.
Early astronomers used solstices (when the sun is at its furthest from the equator) and equinoxes (when the sun crosses the plane of Earth’s equator) as starting and finishing points.
One of the most common ways of measuring the length of a year in ancient times involved the use of a gnomon—a structure that casts a shadow, like the vertical stick or triangle in the centre of a sundial. The shadow cast by the gnomon tracks across the sundial as the sun moves across the sky, and is used to tell the time of day. Sundials were first developed by the ancient Egyptians.
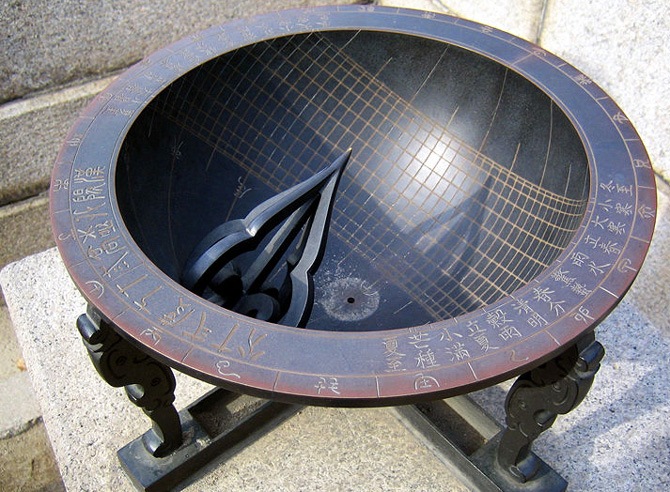
As the shadow cast by a vertical gnomon is shortest at noon on the day of the summer solstice, a count of the days between two summer solstices would give an estimate of the length of the year. This was refined by interpolation between readings on successive days around the summer solstice, and by the construction of ever-larger gnomons, which provided increasingly accurate estimates of the exact time of the solstice. Year lengths were also determined by counting the days between two equinoxes.
Lunisolar
Lunisolar calendars attempted to keep in sync with both the moon and the solar year. This was not an easy task, as there are about 12.368 lunations in a solar year. A lunar calendar consisting of 354 days (12 lunations) would keep in step with the moon—with some days added in from time to time—but would very soon get out of step with the year and, therefore, the seasons.
All calendars were—and still are—plagued by the lack of synchrony between the moon’s cycle and the length of the year, and by the fact that neither the length of the solar year nor the length of the lunar month is a whole number.
The history of the Western calendar
The Roman calendar
The precursor of the calendar in common use today was the Roman calendar. According to legend, it was first used at the time of the founding of Rome, around 750 BCE. It is said to have been invented by Romulus, so is also known as the Calendar of Romulus.
The Calendar of Romulus contained 10 months, starting in March. A complex series of intercalations was required to keep this calendar in step with the moon, the year and the seasons. However, some of the intercalations were at the discretion of certain officials, who, it seems, didn’t always do their job adequately.
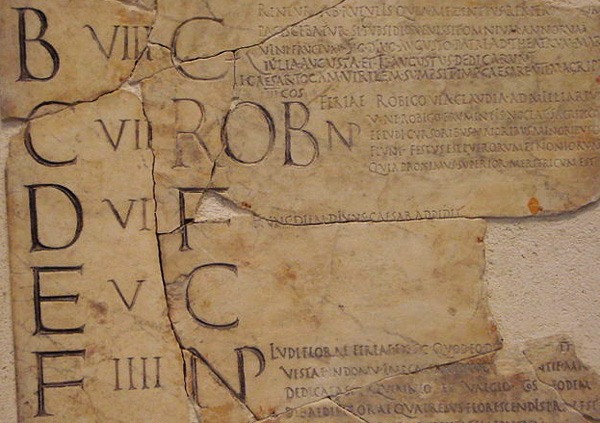
Months of the Calendar of Romulus
Month | Number of days | Origin of the name |
---|---|---|
Martius | 31 | Named for the Roman god Mars |
Aprilis | 30 | Perhaps from the Latin word ‘aperire’, to open, or perhaps from Aphrodite, the Greek name for Venus |
Maius | 31 | Perhaps named for the Greek goddess Maia, or for ‘maiores’, Latin for ‘elders’ |
Iunius | 30 | Perhaps named for the Roman goddess Juno, or for ‘junior’ |
Quintilis | 31 | 5th month—from ‘quin’, Latin for 5 |
Sextilis | 30 | 6th month—from ‘sex’, Latin for 6 |
September | 30 | 7th month—from ‘septem’, Latin for 7 |
October | 31 | 8th month—from ‘octo’, Latin for 8 |
November | 30 | 9th month—from ‘novem’, Latin for 9 |
December | 30 | 10th month—from ‘decem’, Latin for 10 |
Kalendae Nonae Idus
The Roman months were divided into periods of days marked by Kalendae, Nonae and Idus (Kalends, Nones and Ides).
The Kalend was the first day of the month, coinciding with the new moon. The Nonae was the day when the half moon occurred, and fell 8 days before the Idus. The Idus was the 15th day of March, May, July and October, and the 13th day of the other months, and coincided with the full moon. The diagram below illustrates these three milestones, as seen from the northern hemisphere.
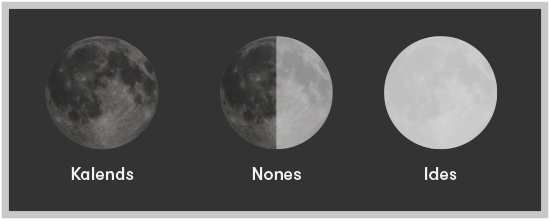
Winter was not assigned any months—it was a separate period of 61 days. Ianuarius (January) and Februarius (February) were later added to the beginning of the year to better align the calendar with the seasons and the year. This had the slightly confusing end result that the months named for their place in the year no longer matched up with their position. For example, December was no longer the 10th month (‘decem’ being Latin for 10), but the 12th.
And even with the addition of Ianuarius and Februarius, the calendar still had some issues, which officials attempted to resolve by including an ‘intercalary month’ in some years. This extra month was added at the discretion of the pontifex maximus (the high priest of Ancient Rome). As the length of political office was determined by the Roman calendar, this gave the pontifex maximus significant political power.
The Julian calendar
By the time of Julius Caesar (100–44 BCE), it had all become quite muddled. Caesar requested a Greek astronomer called Sosigenes to advise him on reforming the calendar. Sosigenes recommended abandoning the lunar calendar and adopting one that focused solely on the solar year. Caesar decreed that each year would consist of 365 days, with an extra day added to every fourth year (this later became known as a ‘leap’ year) in the month of February. To accommodate the change, a once-off adjustment was needed: the year 46 BCE was decreed to be 445 days long—giving some indication of how confused the Roman calendar had become.
The calendar was named in honour of the ruler who began the calendar reform, and the month of Quintilus also became Iulius (July). Sextilis became Augustus (August) in honour of Augustus Caesar, who completed the transition to the Julian calendar during his reign.
The Julian calendar retained the names of the rest of the Roman calendar months.
Months of the Julian calendar
Month | Number of days | Meaning |
---|---|---|
Ianuarius | 31 | From Janus, the Roman god of doors, sunset and sunrise. Janus had one face looking forward, and one looking back |
Februarius | 28, 29 in a leap year | From the Latin word ‘februare’, to purify. The Roman festival of forgiveness of sins was celebrated in this month |
Martius | 31 | From Mars, the Roman god of war. |
Aprilis | 30 | Perhaps from the Latin word ‘aperire’, to open, or perhaps from Aphrodite, the Greek name for Venus |
Maius | 31 | Maia, Roman goddess, daughter of Atlas and mother of Mercury |
Iunius | 29 | Juno, chief Roman goddess |
Iulius | 31 | Previously known as quintilus, ‘5th month’, renamed for Julius Caesar |
Augustus | 29 | Previously known as sextilis, ‘6th month’, renamed for Augustus Caesar |
September | 29 | 7th month—from ‘septem’, Latin for 7 |
October | 31 | 8th month—from ‘octo’, Latin for 8 |
November | 29 | 9th month—from ‘novem’, Latin for 9 |
December | 29 | 10th month—from ‘decem’, Latin for 10 |
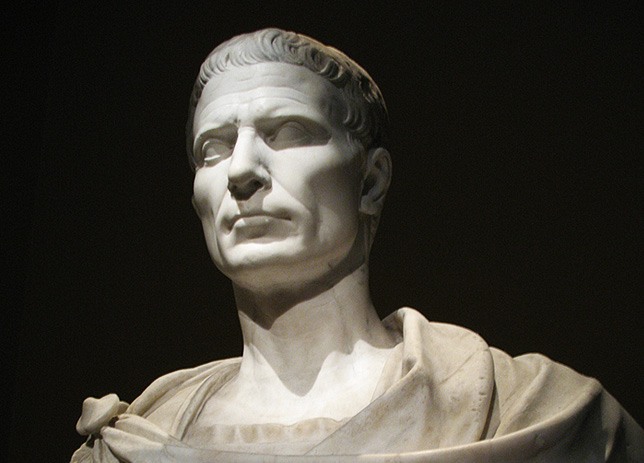
But Caesar’s reform didn’t quite end the confusion. His calendar assumed that each year was 365.25 days long, and that the addition of one extra day every four years would be adequate compensation. However, even then it was known that the actual length of a year was slightly shorter than this—the modern estimate is 365.24219 days. The difference between this and 365.25 is not much—0.00781 days, or about 11.25 minutes. But over time it adds up: in a thousand years, the discrepancy is 0.00781 × 1,000 = 7.8 days.
By the Middle Ages, the Julian calendar was well entrenched in Europe. The system of counting the years since the birth of Christ had been introduced by Dionysius Exiguus, and leap years were deemed to be those divisible by four (the year 1212, for example, was a leap year). But the cumulative error was beginning to be noticed. The vernal equinox, traditionally observed on 21 March, was actually taking place earlier and earlier, and other dates of religious significance were becoming similarly confused.
The Gregorian calendar
Calendar reform was talked about in the Catholic Church for more than 300 years. But it wasn’t until 1582 that Pope Gregory took the advice of mathematicians and astronomers and decreed that the problem would be addressed by omitting three leap years every 400 years. He declared that new centuries would not be leap years unless divisible by 400. This became known as the Gregorian calendar, and is the one we use today.
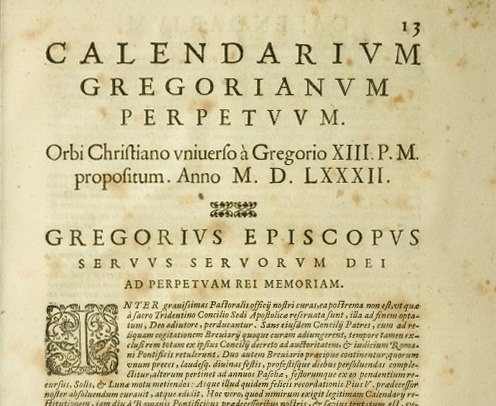
Most European countries adjusted for the accumulated errors of the Julian calendar by omitting 10 days from the year 1582. In fact, people living in what is now Belgium missed out on Christmas because of these cancelled days.
However, Protestant countries largely ignored the decree of the Catholic Pope Gregory. It wasn’t until the 1700s, when the problem of the extra days had become so acute in England, that an adjustment was decreed by parliament. Eleven days were omitted from the month of September in 1752 and Pope Gregory’s system for dealing with century-years was adopted.
The improving accuracy of calendars
Select a calendar type and use the slider to see how many days it is out of step compared to actual days passed.
Atomic clocks
We no longer measure a year by the time between two solstices or a day by the time it takes Earth to complete one rotation on its axis—it’s too imprecise! Earth has a slow wobble in its rotation around its axis, called precession. It’s caused primarily by the moon’s gravitational influence—it acts like brakes on a wheel and gradually slows Earth’s daily spin, making each day ever-so-slightly longer.
So, we now use atomic clocks to precisely measure time. Atomic clocks use a special property of the way a caesium atom holds its 55th (and outermost) electron. A photon of a specific radio frequency is used to knock the electron up to the next highest energy level. Then, when the excited electron relaxes back down to the lower energy level, it kicks out a certain amount of energy. This packet of energy can be thought of as a photon. Caesium is special because its energy levels are remarkably consistent if we’re careful with the radio waves we use to excite that 55th electron. There’s only one excited state and only one lower state, so all the photons it emits have exactly the same energy, which means they have exactly the same frequency: 9,192,631,770 oscillations per second.
Having such a precise way to make a known frequency is useful. We can use it to count time: count to 9,192,631,770 with the sensors in an atomic clock and you have exactly one second. This method is so precise and consistent that every now and again we need to use ‘leap seconds’ to keep the very constant atomic clocks in line with the comparatively inconstant orbit of Earth around the sun.
It’s this incredibly precise measurement that forms the basis of time as we now know it. Sixty of these seconds make an minute; 86,400 seconds make a day; and so on. It’s transmitted out via satellites and mobile phone towers to all our smartphones, keeping them, and us, in sync. The precision is crucial. For example, the signals sent between GPS satellites and receivers on the ground travel at the speed of light (that’s around 30 centimetres every billionth of a second). If the clocks in these systems are out of sync by just one millionth of a second, it can translate to a discrepancy of around 650 metres on the ground in terms of location pinpointing.
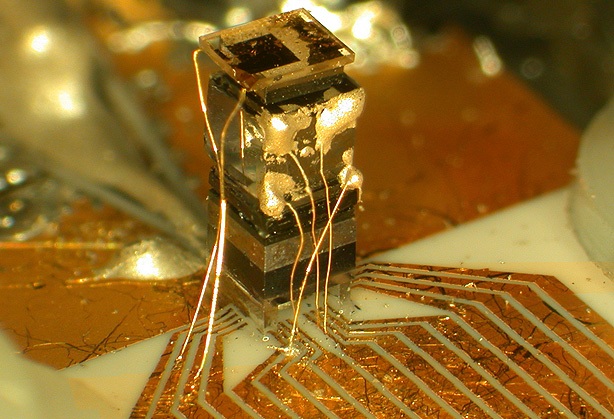
Other cultures, other calendars
Mayan calendar
What’s known as the Mayan calendar was used by several societies throughout what we now call Central America. It comprises three cycles overlaid on top of each other: the Haab, the Tzolkin and the Long Count.
The Haab is a solar calendar, with 365 days, split up into 19 months. Eighteen of the months have 20 days, and one month has just five days. Each month is named with a glyph (picture) that represents a personality trait connected to that month. Each day is documented with a number, followed by the glyph for the month.
The Tzolkin cycle has 260 days, divided up into 20 periods of 13 days each. Each day is represented by a glyph. The Tzolkin is the sacred calendar, used for keeping track of religious events.
The Long Count, known as the ‘universal cycle’, covers much longer periods of time, with each cycle comprising 2,880,000 days. The Mayans believed that the world was destroyed and created anew at the end of each Long Count cycle.
The three cycles are arranged in wheels. There is a smaller wheel, with 260 positions, for the Tzolkin cycle and a larger one, with 365 positions, for the Haab. The wheels rotate in different directions, and, for any given day, the Tzolkin day will align with a day in the Haab cycle. Together, these are known as the Calendar Round, which takes around 52 years to complete.
The Long Count is divided up into kin, uinal, tun, katun and baktun.
Division name | Period |
---|---|
kin | 1 day |
uinal | 20 days (20 kin) |
tun | 360 days (18 uinal) |
katun | 7,200 days (20 tun) |
baktun | 144,000 days (20 katun) |
Long Count cycle | 2,880,000 days (20 baktun) |
The date notation was given in the form: baktun.katun.tun.uinal.kin—so 3.17.6.0.9 would indicate 3 baktun, 17 katun, 6 tun, zero uinal, 9 kin. Obviously, the Mayans would use their number glyphs, not our numerals!
See what today’s date looks like at the Smithsonian Living Maya Time website's calendar converter.
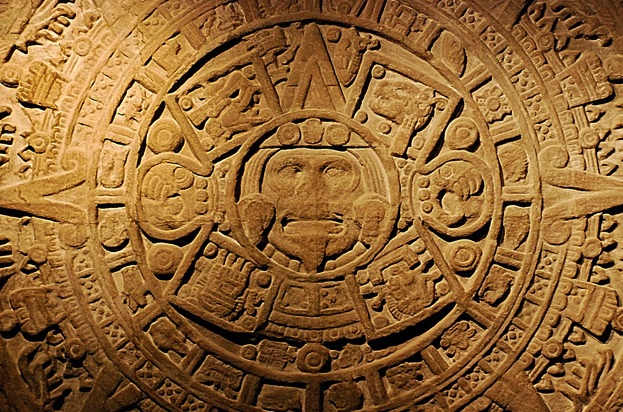
Chinese calendar
There is evidence—inscriptions on what are known as Shang oracle bones—that the Chinese were using a calendar based on a solar year of 365.25 days as far back as the 14th century BC. China used the traditional Chinese calendar until 1912—the Gregorian calendar only became widely used after the Communist Party came into power in 1949.
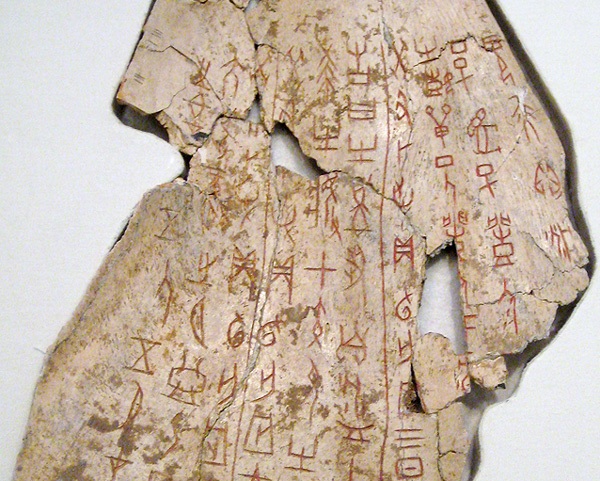
A lunisolar calendar, the Chinese calendar incorporates observations of the sun and the phases of the moon. Years are determined by the sun, and months are set by lunations. An ordinary year has 12 months (353–355 days), and leap years have 13 months (383–385 days).
Each year in the Chinese calendar has a twofold name—with a ‘celestial’ component and a ‘terrestrial’ component. The names of the years cycle through the celestial and terrestrial sequences, and a full cycle takes 60 years. It is thought this naming system began in the year 2637 BC.
Celestial branches
Terrestrial branches
See what today's date looks like in the Chinese calendar on the Prokeraia website's calendar converter.
Islamic calendar: Hijri Qamari
The Hijri calendar is a lunar calendar, with its first day being the day that Mohammed travelled from Mecca to Medina, the event known as the Hijra. This day was 16 July, 622 AD (on the Julian calendar). Being a lunar calendar, it’s around 11 days shorter than the Gregorian calendar. It has 12 months, each beginning with a new lunar cycle.
Some Muslims rely on a visual sighting of the new moon phase to start a new month, while in other parts of the world, a new month begins on days calculated by astronomical projections of the moon phases. As they’re based on the lunar cycle, months have either 29 or 30 days, and their starting day can vary according to the method used to mark the new month.
The Hijri is used to observe all the significant occasions of the Muslim faith, including Ramadan, the month of fasting; the time of Hajj, the pilgrimage to Mecca; and other festivals and important events.
See what today's date looks like in the Islamic calendar on the Fourmilab website's calendar converter.
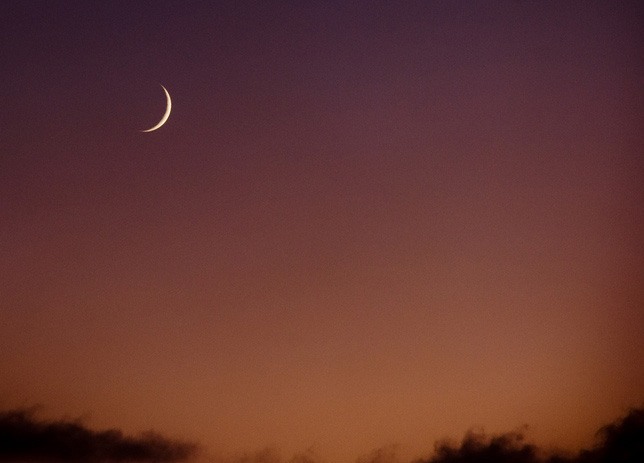
Indigenous Australian calendars
Indigenous Australians also used astronomical movements, particularly of stars or certain constellations—often their heliacal rising, which is the time when they are first visible on the eastern horizon just before sunrise—to track time and signify events.
The rising of the Pleiades constellation in the dawn sky indicates the beginning of Nyinng (the cold season) for the Pitjantjatjara people of southern central Australia. The Kaurna people of the Adelaide Plains recognise a number of seasons according to certain stars or constellations: the beginning of Parnatti (the autumn rainy season) is marked by the appearance of the ‘Parna’ star, which indicates rains are coming and it’s time to build strong waterproof shelters. Willutti (spring) is marked by the eagle star ‘Wilto’, and Woltatti (the hot season) by ‘Wolta’, the bush turkey constellation.
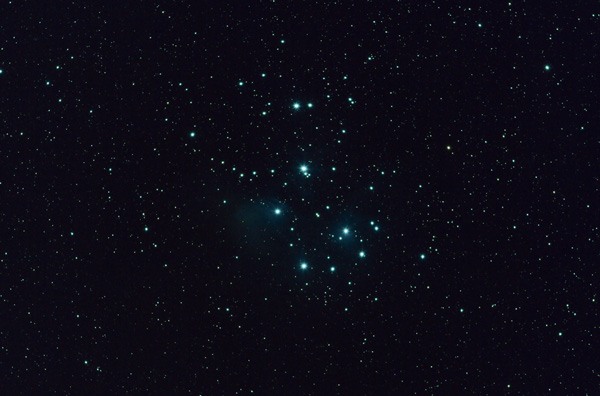
When the malleefowl constellation (Lyra) appears in the night sky during March, it indicates to the Boorong people of north-western Victoria that malleefowls are building their nests. By the time the constellation disappears in October, eggs will have been laid in the nests and can be collected.
Some ceremonies are also conducted according to the timing of astronomical events. The Yolngu people of Arnhem Land, in the Northern Territory, track the path of Venus through the sky, holding their ‘Morning Star’ ceremony at times when Venus rises before dawn.
There are other calendar systems based on observations of weather and changes in climate, the flowering and fruiting of plants and the behaviour of animals within the environment in which people live. This makes perfect sense in a society that depends upon and manages its environment, where observations of how the landscape and the food sources change over time are essential for survival.
Seasons are marked by events relevant to people’s everyday life—the abundance of a particular food source, such as shellfish, fruits, eggs or berries, or the appearance of particular flowers that act as a cue to target particular animal species.
Just one example of this is the calendar documenting traditional knowledge from the Tiwi Islands, north of Darwin in the Northern Territory. This seasonal calendar is split up into three major seasons, with 13 minor overlapping shorter seasons.
Tiwi major seasons
Season | Description |
---|---|
Kumunupunari | Dry season of fire and smoke. (March–August) |
Tiyari | Season of hot weather and high humidity. (September–November) |
Jamutakari | Wet season when pakitiringa (rain) falls consistently and the swamps, creeks and rivers are full. Wunijaka, the north-west wind blows and brings rain. There is much pumurali (lightning) and thunder with the rain. (December–February) |
Tiwi minor seasons
Season | Description |
---|---|
Wurringawunari | Season of the knock-em-downs. This is the first part of the dry season when winds blow from the south-east, flattening the tall grass and drying up surface water. |
Kimirrakinari | Season of fire; dry grass is burnt. |
Pumutingari | Season of wind that flakes the skin. |
Yirriwnari and Mirniputari | Season of cold weather in the middle of the dry season is signalled by the flowering of Wurritjinga (Eucalyptus confertiflora). |
Kumwari | Season of fog. Temperatures are low; mornings are foggy. |
Yartupwari | Season of the dry creek bed. Waterholes and creeks dry up. |
Milikitorinari | Season of hot feet. The hot ground burns the soles of the feet. Food gathering is mainly among mangroves and jungle patches rather than on dry plains and in woodlands. |
Pumwanyingari | Season of thunder. Humidity is high, afternoons are cloudy, and there is little rain. |
Kurukurari | Season of the mangrove worm. Worms are abundant, sweet and filling. |
Mumpikari | Season of muddy possum tracks. With the first rains, muddy tracks of possums are seen as they return to their trees after foraging on the ground, making them easier to track and hunt. |
Tawutawungari | Season of the clap sticks. Kurlama (special yam) ceremonies are held. |
Wurrijingari | Season of flowers. |
Marrakatari | Season when tall grass flowers. This indicates end of the wet season. |
Recording traditional knowledge
CSIRO has worked with this Tiwi Islands community and various other Indigenous groups around Australia to document and record their traditional knowledge of the seasons, creating pictorial calendars. With their deep associations with the local climate, flora and fauna, these systems have direct relevance, and often a more practical application, than the comparatively arbitrary notion that the month of September has 30 days, after which we move on to October.
CSIRO research with Tiwi Islanders to document and record their traditional knowledge of the seasons and the environment was supported by an Inspiring Australia Unlocking Australia’s Potential grant. CSIRO also worked with a number of other Aboriginal language groups across northern Australia to create seasonal calendars, as part of the Tropical Rivers and Coastal Knowledge and National Environmental Research Programs. The calendars reveal the annual cycles of meteorological events, water flow, lifecycle stages of plants and animals, and key environmental indicators. This rich phenological knowledge is a key driver of people’s behaviour, particularly the harvesting of bush resources and has more practical application than the comparatively arbitrary notion of dates on a calendar.
We think of time as something constant, inexorable. But the ways we measure it are hugely influenced by our culture and religion—a calendar is the result of a complex interplay between the underlying science of the astronomy, climate, or natural environment on which it is based, and the social fabric of the society that uses it.