Brain games: how athletes' minds work
Expert reviewers
Essentials
- Athletes have excellent cognitive skills at the level of perception, anticipation and decision making.
- A bank of memories of similar moves and experiences, and a pattern-based mode of memory retrieval, helps expert sportspeople make quick decisions.
- Mirror systems in athletes’ brains could contribute to their ability to accurately predict the moves of other players.
- Practice can change the brain, strengthening neural connections and even altering its structure.
- Scientists continue to debate the relative importance of natural talent versus practice when it comes to explaining athletes’ abilities.
The broad and muscled shoulders of an Olympic swimmer, the sleek and toned legs of a professional cyclist, the slim yet powerful physique of a champion gymnast … it’s pretty obvious that the bodies of elite athletes are different to the rest of us. Their impressive physiques, honed to perfection, can jump higher, move faster and throw harder than many of us could ever dream of. But it’s not just their amazing bodies that set these elite performers apart—it’s their brains, too.
Inside the mind of the elite athlete
Quicker thinkers?
It’s clear that elite sportspeople have outstanding hand–eye coordination. We’ve all observed how expert tennis players, for example, seeing an oncoming ball, can move quickly to meet it with their racquet. But, as we marvel at their lightning-fast bodies, it can be easy to forget what links the eye and the hand. That’s right—it’s the brain. In elite sportspeople, the brain processes for rapidly perceiving information specific to their sport, such as the movement of an opponent or the trajectory of a rapidly approaching ball, and making the correct movement, are (thanks to many hours of practice) very highly developed.
As well as excellent hand–eye coordination, expert sportspeople also have the ability to make split-second predictions and decisions. Take cricket, for instance. Once the ball leaves a fast bowler’s hands, the batsman may have less than a second to get his body and bat in the right position to meet the assault. With the ball flying towards him at speeds of up to 160 kilometres an hour, he’ll need to do some pretty fast footwork. But a quick body isn’t enough—in order to figure out where the ball is likely to go, and where he’ll need to be to meet it with his bat, he’ll need a quick mind, too.
Indeed, it turns out that a quick mind is an important factor in giving elite athletes the edge. Experiments have shown that sportspeople at the top of their game are able to predict which way an object is likely to travel earlier than novice, and even intermediate, athletes, giving them more time to plan an appropriate action in response—essential in fast-moving ball sports such as cricket, tennis, soccer or basketball.
In an experiment with cricket players, for example, experts, intermediate players and novices were shown footage of an oncoming swing (fast) bowler. The footage was stopped at various points as the bowler advanced, and subjects were asked to predict whether the ball would swing into or away from the body of a right-handed batsman. It was found that expert players could more accurately predict the trajectory of the ball much earlier than the intermediate or novice players—and their predictions continued to improve as more of the film was shown.
In another experiment, subjects were shown video images of a swing bowler about to release the ball. Parts of the bowler’s body were removed, and subjects asked to predict which way the ball would go. Researchers discovered that, in order to predict the trajectory of the ball early in the bowler’s advance, experts needed to be able to see the bowler’s arm and hand—indicating that, for elite cricket players, wrist angle is an important visual cue.
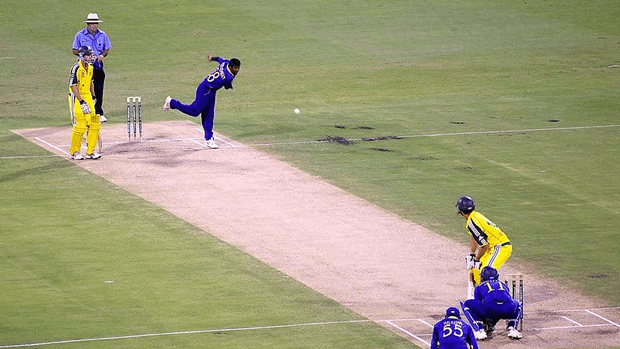
The excellent anticipatory abilities of elite players in time-stressed sports aren’t limited to cricket players—they’ve also been demonstrated for a range of other ball sports including tennis, volleyball, soccer and basketball. As we’ve seen, these expert players rely on postural information from their opponent in order to anticipate what’s coming next. It’s the combination of these postural cues with situational information (such as the field setting, previous encounters against that bowler, and so on) that feeds into these players’ excellent anticipatory performance.
Better memories?
Expert athletes’ ability to make predictions may also be built on their capacity to remember the past—that is, their memories. Through practice and experience, expert sportspeople build up a huge store of what are called ‘procedural’ memories. These are not conscious, but are based on the body’s memory of actions and events which they’ve previously encountered. It’s the type of memory which enables our body to know what to do to ride a bicycle without us having to think about it. But this memory isn’t just about muscles or body movements—it also stores things like the way a ball moves or turns when approaching us, or the way an opponent’s racquet swings to send a ball left or right. The brain uses these memories to automatically make predictions and, the more experience or practice we have, the more accurate those predictions are. Elite sportspeople’s brains automatically draw on these memories to make highly accurate predictions about the move an opponent will make or the direction a ball will go in a fraction of a second, allowing lightning quick and highly precise reactions.
It also appears that a pattern-based mode of memory retrieval helps experts make quick, anticipatory decisions. As expertise develops, experts learn to ‘chunk’ information. So, rather than seeing nine other players running around them in a game, an expert basketball player will perceive specific offensive or defensive pattern—and consequently will have a fair idea what the outcome of that pattern will be: where the players will move, who should get the ball and so on. This single ‘chunk’ of information can be retrieved much more easily—and, importantly, more quickly—from the player’s memory than nine separate pieces of information relating to each player.
Expert mirror systems
It was thanks to a monkey, a peanut and a bit of good luck, that scientists in Italy discovered what are now known as ‘mirror neurons’. The researchers were studying the brains of macaque monkeys: by placing electrodes in a monkey’s premotor cortex (the area of the brain which directs muscle movement), they were able to observe which neurons fired when the animal reached for a peanut.
But it was when a person reached for a bit of nutty goodness that the scientists discovered something even more interesting. The same motor neurons that had fired when the monkey had reached for a peanut also fired when the monkey watched someone else reaching for a peanut! As far as the monkeys’ brains were concerned, watching someone doing something was the same as doing it themselves.
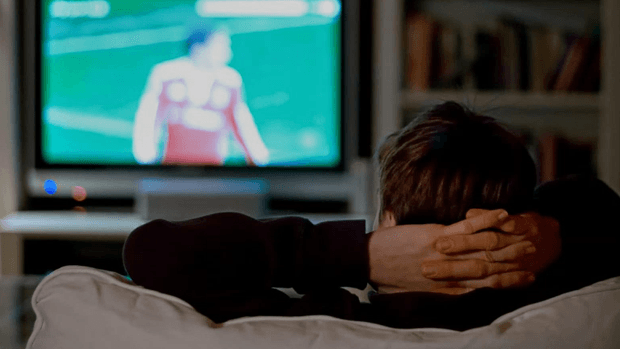
The same, evidence suggests, is true for people—our brain ‘mirrors’ what it sees. In our premotor cortex, about a fifth of the neurons that fire when we perform an action (such as kicking a soccer ball) will also fire when we see someone else doing that action (like when we’re watching someone kick a soccer ball on TV). But it appears that, when it comes to a sport in which they’re an expert, elite athletes’ mirror systems may be just that much more precise.
Researchers looked at professional basketball players, expert basketball observers and non-experts to see what went on in their brains as they watched movies of basketball players taking free shots. As they watched the footage, the responses of each of these groups’ muscles to electrical stimulation of the motor cortex were observed. Both the expert watchers and the expert players showed an excitability of brain areas relating to movement as they watched the shot. But it was what they found in the brains of the expert players that was particularly intriguing. The activity in the brains of expert players, especially when watching shots that wouldn’t make it into the basket, was specific to the particular hand muscles which determined the fate of the shot.
This mirroring in the brain of another player’s body movements may enable expert athletes to create forward models to predict the outcome of those movements—allowing them to respond in a split second. The action of mirror neurons may also explain why watching an action as part of training or coaching (observation-based learning) can help athletes learn how to do it better.
The increase in brain activity relating to particular actions, like hand movements, seems to be restricted to those actions that the athlete, in the course of playing their chosen sport, has actually done themselves. So, sadly, even with the benefit of mirror neurons, being a professional couch potato won’t make you a professional soccer player!
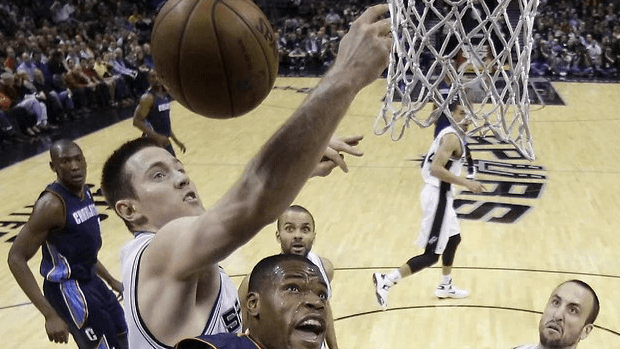
While watching a free throw taken by another player, basketball players’ brains precisely mirrored that action—down to the specific hand muscles. Image source: worldbasketusa / Flickr.
Brain training: how practice changes the brain
Athletes train a lot. And I mean a lot. Elite swimmers, for example, can spend up to six hours a day in the water. The results are visible in their body’s speed and agility, and in their physique—swimming muscles strengthened and toned from many sessions in the pool. But practice not only changes athletes’ bodies; it also changes their brains.
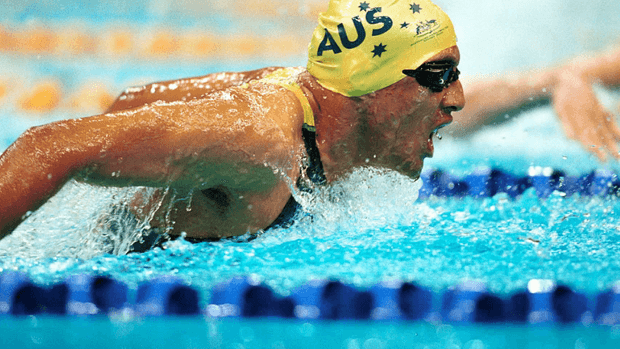
While human brain development is over by around the age of 25, our brains continue to change throughout our lives. Depending on our experiences (including the memories we revisit and the skills we practise) some synaptic connections (connections between neurons) become stronger, while others wither away. This ability of our brains to change is known as plasticity.
You can think of the connections between neurons like pathways in your brain. As an analogy, imagine you walk through a field of long grass each day to get to the bus stop. At first, wading through the overgrown greenery is a struggle. The more times you walk that way, however, the flatter the grass becomes, making a pathway that’s easier and easier to travel along. Likewise, learning a new skill can be hard at first. But the more we do something, the stronger the neural pathways associated with that skill become, and the easier and quicker the task. If we practise enough, we may even begin to run on ‘autopilot’—with our actions becoming automatic and unconscious. And, as you’ll know if you’ve ever ‘overthought’ a tricky dance or sporting move, interfering with this unconscious process can actually worsen performance.
So, what does the move towards greater automaticity look like in the brain? One study looked at the brains of novice, expert and elite archers performing a simulated archery task. The novices showed widespread brain activity when performing a simulated archery task, especially in a number of frontal areas of the brain related to controlled planning. Experts, by contrast, showed reduced activity in these areas; as an activity becomes more automated and less controlled, they appear to rely on specialised processing in particular areas in the brain (including the cerebellum). This reduction of general, widespread activity in the brain in favour of specialised, localised processing may be what makes it possible for expert athletes to make decisions quickly, efficiently and using less energy than novices.
Practice can even change the structure of the brain. One famous study looked at the effect of practice on the brains of London cab drivers preparing for the fiendishly difficult test of the city’s geography known as the ‘Knowledge of London’. It found that the posterior part of the hippocampus (an area especially important for spatial memory) was enlarged in these drivers—and it became bigger the longer they did their job.
A similar thing happens when people learn new motor skills. People learning to juggle, for example, show growth in the amount of their brain’s grey matter GLOSSARY grey matterthe darker tissue of the brain and spinal cord, consisting mainly of nerve cell bodies and branching dendrites over time, with increases in the brain’s motion-sensitive middle temporal area. Interestingly, this structural growth seems to reverse when practice ends, even though performance levels remain high. A study of professional divers found that the cortex of certain areas of their brains became thicker the more years of practice they had—especially the area known as the parahippocampal gyrus, important for acquiring spatial information. Expansion in the cerebellum, the part of the brain which coordinates and regulates muscular activity, has also been observed in rock climbers, basketball players, badminton players and short-track speed skaters.
Mental endurance
Ever noticed how elite athletes can keep focused for so long, able to keep pushing their body even when almost completely exhausted? Recent research provides some clues about how they’re able to do this. Just like our muscles, the brain needs fuel to work. Experiments in rats show that endurance performance depletes fuel stores in regions of the brain. However, after endurance training, these fuel stores in the brain grow—with the rats able to last longer the next time they go for a spin on the treadmill.
Assessment of these characteristics hasn't yet been done in humans, but it’s thought that such fuel may be important for ongoing attention and motivation, as well as other brain actions. This is one possible explanation for endurance athletes’ superior physical performance after a prolonged cognitive task that recreational athletes found exhausting. The changes in brain efficiency and structure which we looked at earlier may also preserve performance by being less of a drain on the brain’s resources.
Faster, harder, smarter?
As we’ve seen, expert athletes don’t just run faster or throw harder to excel at their sport—they also use superior higher-order planning and perceptual skills. Thanks to those long hours of training and practice, their brains may even be physically different from ours—altered by practice just as surely as their muscles have been.
But, when it comes to mental processes, are elite athletes generally just sharper? Do their superior cognitive skills extend beyond their chosen sport? The answer seems to be that it depends on which cognitive abilities you’re talking about. Experiments with tennis players, for example, found that they were better than non-players at detecting the difference of the speed of dots moving towards them. When it came to finding tennis balls in a visual scene, however, they performed better than non-players only when the scene was a tennis snapshot; when asked to find tennis balls in a sport scene not related to tennis, they were no better than the non-players. The memory abilities we looked at earlier are also specific to the expert’s particular domain—a tennis player’s bank of tennis moves stored in their long-term memory GLOSSARY long-term memoryLong term memory consists of both explicit memory (when we consciously remember something) and implicit (or unconscious) memory. Implicit memory includes memory relating to how to carry out learned motor skills, such as riding a bike. probably isn’t going to help them much in a basketball game, for example.
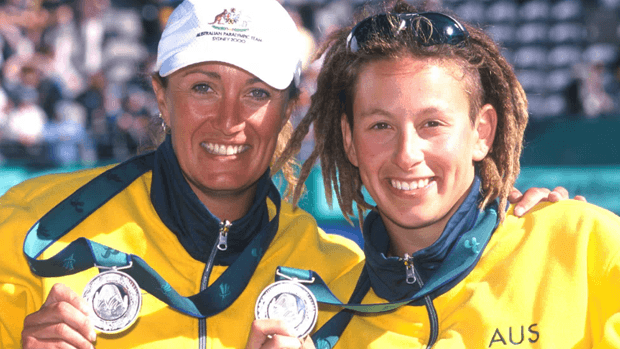
As to the relative importance of natural talent versus deliberate practice … well, that’s something sport scientists will no doubt keep thrashing out. In the meantime, why not drag yourself away from the screen, pull on some sports shoes, and start learning some new sporting skills? You won’t just be giving your body a workout—you’ll also be exercising your brain.