Getting our head around the brain
Expert reviewers
Essentials
- The human brain is the most complex organ in the human body.
- The brain constitutes 2 per cent of a person’s body weight but consumes 20 per cent of the body’s energy.
- A healthy adult brain contains approximately 86 billion neurons.
- It is a myth that we use only 10 per cent of our brains—every part of the brain has a known function.
- Human brains are wrinklier than the brains of any other animal—a key factor in our higher intelligence levels.
Understanding how the human brain works gives us an insight into what makes us human. If an animal has a backbone it also has a brain. Elephants, dogs, lizards, frogs, and even fish, have brains. But none of these creatures demonstrate the same capacity for learning, language, emotion and abstract thought as the human species.
What is it about the human brain that gives us the edge? It’s not the size—many animals have larger brains than we do. While neuroscientists and philosophers have studied the functioning of the brain for hundreds of years, there are aspects of our brainpower that remain among humanity’s most enduring mysteries.
An introduction to the brain
The basic facts about the brain are well known. Weighing in at around 1.3 kilograms, it is one of the largest organs in the human body. It sits inside the skull, immersed in a fluid that cushions it from sudden impacts to the head.
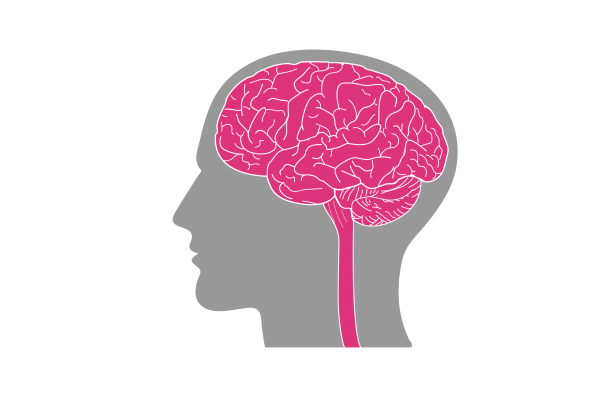
It is nothing remarkable to look at—a wrinkled object about the size of a number 13 chicken—but it consists of a complex and intricately tangled mass of nerve cells, called neurons.
Neurons are the basic units that make up the brain and nervous system. They are specialised cells that act like telegraph wires carrying messages in the form of electrochemical impulses throughout the body. These impulses travel very quickly: it takes about a hundredth of a second for a pain in your little toe to register in your brain. This is quite remarkable, given that the impulse travels a complex path through many neurons and across the gaps (synapses) between neurons to reach its destination. It is estimated that the adult human brain contains about 86 billion neurons.
Brain functions
The brain performs a number of functions, many of which are related to the physical needs and actions of the body. For these functions, the brain can be thought of as the command centre of the human nervous system, much like the headquarters of a military unit. It receives information from its vast network of neurons throughout the body. Based on this information, it makes decisions and issues commands that stimulate muscles and give the body movement. These are both unconscious (breathing, keeping the heart beating, or analysing sound) and conscious (turning our head, picking up a toy, or speaking). Interestingly, movements and speaking can also be considered to be ‘unconscious’. For instance, playing the violin, changing gears while driving, or walking while talking can be automated, particularly as you become experienced. This is like functioning on autopilot.
Other brain functions are more like those of a university than a military headquarters. These functions give us the ability to read, write, talk, learn, express emotion, create art—in essence, they give us our humanity.
Structure of the brain: an overview
The brain is shaped like two fists standing side by side on a single wrist. The ‘wrist’ is the brain stem, connecting the brain to the spinal column, and the ‘fists’ constitute the left and right hemispheres of the largest part of the brain, the cerebrum. At the back of the brain, below the cerebrum, is the cerebellum. Within each of these parts are specific regions that control specialised functions. Let’s take a look at each section in a bit more detail.
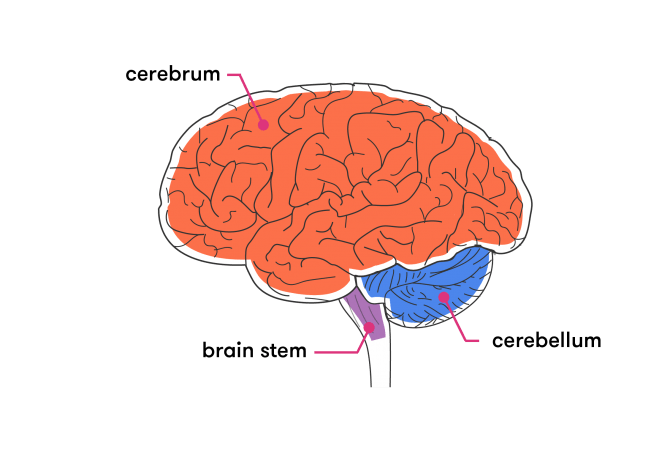
The cerebrum
The cerebrum is the largest and, for most people, the most easily recognisable part of the brain. From an evolutionary perspective, it is also the newest part. It’s in this section that processes such as perception, decision-making, thought, judgement and imagination occur.
The cerebrum has an outer layer known as the cerebral cortex. The largest part of this layer is known as the neocortex (Latin for ‘new bark’), and is composed of six thin layers of neuron cell bodies and non-neuron brain cells called glial cells (more on these later). Their slightly grey colouring is what gives this part of the brain the term ‘grey matter’. The cerebral cortex is arranged in distinctive folds.
These ‘folds’ or wrinkles serve an important purpose. They effectively increase the surface area of the brain, thereby increasing the number of neurons within it and, at the most basic level, more neurons = more connections = a more efficient brain. In fact, the cortex is so heavily folded that if you were to spread it out, it would cover 0.23 square metres. Human brains are wrinklier than the brains of any other animal, a key factor in our higher intelligence levels.
Despite being just a few millimetres thick, the cerebral cortex constitutes 40 per cent of the entire brain mass.
A deep channel divides the cerebrum into two unique halves, or hemispheres (often colloquially referred to as the left and right brain), which communicate with each other via a band of nerve fibres called the corpus callosum. The right side of the brain controls movement in the left side of the body and the left hemisphere controls the right side of the body.
The two halves work closely together—while there are functions that are considered more left than right brain, and vice versa, the jury it still out on how much specific functions are lateralised, and how uniformly across individuals. The left hemisphere is generally considered responsible for the control of analytic functions, such as speech and language, while the right tends to be associated with facial recognition, spatial orientation and the ability to recognise patterns. However, many neuroscientists believe that language is the only function that can be confidently said to be predominantly one side (left) over the other.
Lobes
Each cerebral hemisphere is divided up into four regions called ‘lobes’: the frontal lobe, parietal lobe, occipital lobe and temporal lobe.
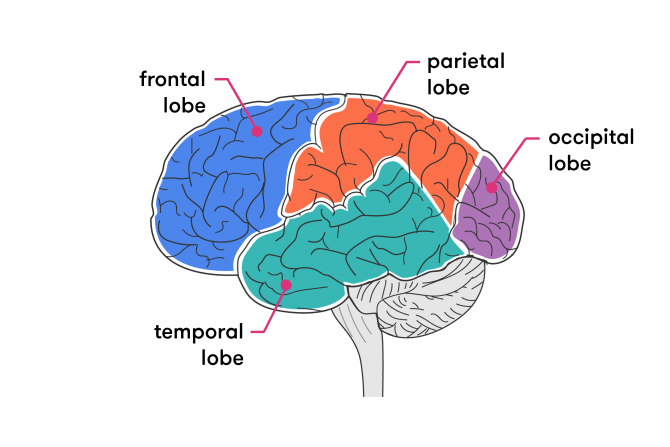
While lobes each play specific roles in human thought and activity (listed below), they do not work in isolation. The lobes are strongly connected with each other, as the brain functions within networks rather than in isolated ‘modules’.
- frontal lobe
- reasoning, planning, parts of speech, movement, emotions, problem solving
- parietal lobe
- movement, orientation, recognition, perception of stimuli
- occipital lobe
- visual processing
- temporal lobe
- perception and recognition of auditory stimuli, memory, speech and ‘theory of mind’ (understanding the minds of others).
Subcortical structures
The cerebrum also contains several subcortical GLOSSARY subcorticallocated in the region of the brain below the cortex structures, including the hippocampus (involved in memory function), the basal ganglia (involved in coordination of movement) and the olfactory bulb (involved in sense of smell).
Humans have the largest cerebral cortex (relative to the size of their brains) of all mammals. Because this section of the brain is responsible for the ‘higher functions’, such as memory, communication and rational thought, it’s believed that we owe our intelligence, and difference from other animals, to our highly developed cerebral cortex.
The cerebellum
The cerebellum is found at the back of the brain near the base, tucked beneath the cerebral hemispheres. Sometimes referred to as the ‘little brain’, it contains both grey matter (made up of cell bodies, dendrites and axon terminals of neurons) and white matter (made mostly of glial cells and myelinated axons GLOSSARY myelinated axonsAxons surrounded by a fatty white substance known as myelin. Myelin is essential for the proper functioning of the nervous system. ). The cerebellum works to transmit information to and from the spinal cord and to other parts of the brain.
Specifically, it is known to be responsible for the maintenance of balance and posture, coordination and regulation of muscle movement and activity, and motor learning such as precision and accuracy in catching and hitting a ball. Damage to the cerebellum affects many of these skills—and it’s for this reason that it has been traditionally considered a motor system. It does not initiate motor commands, but modifies and transmits the descending signals to make them more accurate. It is important in allowing us to perform everyday voluntary tasks, such as writing and walking.
However, studies conducted during the 1990s showed that the cerebellum has roles beyond motor control. It is also involved in various cognitive functions, such as language, memory, social cognition, attention and emotion, though its role in these additional functions is not yet well understood. Evolutionary biologist Robert Barton from Durham University in England said that the findings of recent studies on the role of the cerebellum may ‘shift attention away from an almost exclusive focus on the neocortex as the seat of our humanity’.
Another important property of the cerebellum is its ability to learn and remember, which scientists believe is based, among other things, on the distinctive cell architecture of the cerebellar cortex.
Physically, the cerebellum is the second-largest part of the brain. Although accounting for only 10 per cent of the brain’s volume, it contains over half of the total number of neurons (specialised cells that transmit information via electrical signals). Like the cerebrum, the cerebellum is also made of two hemispheres, though its folded outer layer (cerebellar cortex) has smaller, finely spaced parallel grooves as opposed to the larger, more irregular folds of the cerebral cortex.
From an evolutionary point of view, the cerebellum is the older part of the brain. It is not unique to humans, and is present in animals that existed prior to Homo sapiens.
The brain stem
The brain stem connects the brain with the spinal cord and, although it is one of the most basic parts of the brain, it’s one of the most important for ensuring the body’s survival, controlling basic bodily functions such as breathing, swallowing, consciousness and blood pressure. Any damage to the brain stem is usually serious and life threatening.
The brain stem is a tube-shaped mass of nervous tissue, measuring around 8 centimetres in length, which, as it ascends from the spinal cord, becomes more complex in both its internal and external structures.
It is situated at the base of the brain, above the spinal cord and below the cerebrum, and has three primary functions: 1) it relays information from the brain to the body and vice versa, via the brain stem, 2) it gives rise to cranial nerves, and 3) it has integrative functions including control of the cardiovascular system, pain sensitivity, respiration and consciousness.
The brain stem consists of the midbrain, pons and medulla.
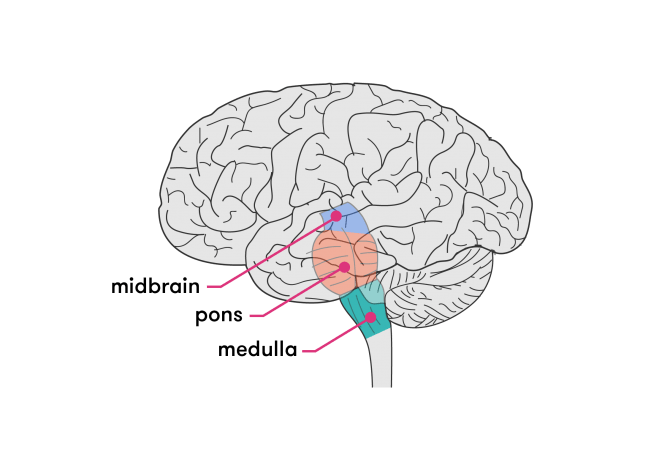
Midbrain
The midbrain, also called the mesencephalon, is the portion of the brain stem that connects the hindbrain (consisting of the cerebellum, the pons and medulla) with the forebrain (cerebral cortex). Its functions include eye movement, sight response, pupil dilation and body movement. It also regulates autonomic functions (those that the body carries out without conscious thought) such as digestion, heart rate and breathing rate.
Pons
In Latin, ‘pons’ means ‘bridge’. The pons plays a critical role in communication, containing all the neurons that connect the higher regions of the brain to the medulla and spinal cord. The pons also connects the left and right sides of the brain to each other, as well as connecting the cerebellum to other regions of the brain. The pons contains nuclei that deal with functions such as sleep, breathing, swallowing, bladder control, hearing, equilibrium, taste, eye movement, facial expression, facial sensation, and posture.
Medulla
The medulla oblongata is located in the lower portion of the brain stem and contains all of the neurons that connect the brain to the spinal cord. It is here that about 90 per cent of these neurons switch from the left side of the body to the right, and vice versa. As neurons pass through the medulla they also form many relays, where one neuron passes its signal on to another neuron that continues onward to the brain or body. The medulla contains the cardiac, respiratory, vasomotor and vomiting centres, and deals with involuntary functions such as blood pressure, breathing and heart rate.
How does it all work together?
So we now know about the different parts of the brain, but what makes them all work together with our bodies?
Neurons
As we mentioned earlier, neurons are the basic units that make up the brain and nervous system. They are specialised cells that act like telegraph wires carrying messages in the form of electrochemical impulses throughout the body. The brain is made up of approximately 86 billion of these nerve cells, with close to a quadrillion connections between them. It is the ability of these neurons to gather and transmit electrochemical signals that keeps our brain firing.
Neurons vary so much in shape that it isn’t possible to describe a ‘typical’ one, but they do have three major features in common.
- cell body
- Each neuron has a body (or soma), containing a nucleus, endopalsmic reticulum and other material to keep the cell alive.
- axon
- This long, cable-like projection of the cell carries the electrochemical message/instructions along the length of the cell.
- dendrites
- One or more (usually numerous) fine, branching extensions. They make connections to other cells, and are used to send and receive information. Dendrites can be found on one or both ends of a cell.
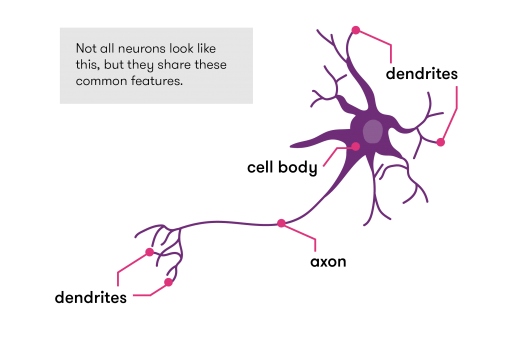
Transmission of a nerve impulse
The electrochemical aspect of neurons lets them transmit signals over long distances (up to a few metres) and send messages to each other.
When activated, neurons transmit a wave of electrochemical charge. This wave of charge is called an impulse. The starting point of an impulse could be a sense organ such as the skin, an eye, an ear, the nose or the tongue, or it could be at a dendrite that has received a message from another neuron. When a neuron is stimulated, it transmits the impulse electrically along its axon. At the end of the axon the impulse travels across a tiny gap, called a synapse, to another neuron (or to a gland or muscle) by means of special chemical messengers called neurotransmitters.
The neurotransmitters affect the next cell in one of two ways: they either ‘excite’ it, so that it will send the impulse to the next cell, or they ‘inhibit’ it. The neurotransmitter molecules either break down or are reabsorbed after they have delivered their ‘message’.
The electrical transmission of a nerve impulse is basically the same in all instances. But at the junction between cells, the chemical transmission of the impulse provides the capacity for differentiating between messages.
In very simple connections in the nervous system, a single string of neurons is arranged end to end, with the axon of one ending on a dendrite of the next. Usually the connections are more complicated than this: a single neuron may have as many as 20,000 connections to other neurons. Not all of the connections in the nervous system are between two neurons—they can also connect to muscles or glands.
Glial cells
Neuroglial cells, generally referred to simply as glial cells or glia (from the Greek, meaning ‘glue’) are very different from nerve cells. For many years it was believed that glial cells were passive bystanders in neural transmission. However, recent research has indicated that glial cells in several areas of the brain, such as the hippocampus and cerebellum, do participate in synaptic transmission, as well as having other functions.
While this area of research is still being investigated, we do know many of the other roles that glial cells (of which there are five types) play. They maintain homeostasis GLOSSARY homeostasisthe property of a system in which variables are regulated so that internal conditions remain stable and relatively constant , form myelin GLOSSARY myelina mixture of proteins and phospholipids forming a whitish insulating sheath around many nerve fibres, which increases the speed at which impulses are conducted , work to maintain the signalling abilities of neurons—including supplying them with nutrients and oxygen—and provide neurons with protection and support. They can also create a scaffold for some aspects of neural development, and can aid in (or prevent, in some instances) recovery from neural injury.
Glial cells outnumber nerve cells in the brain by around three to one, and although they have complex extensions protruding from their bodies, they lack axons and dendrites, and are generally smaller than neurons.
Although glia are the poor cousins of neurons when it comes to media attention, they are vital for a healthy, functioning brain. Without them, neurons simply would not work properly, and could not do the job that they do.
How do we study the brain?
It’s not so long ago that the only way scientists could study the human brain was to dissect it after a person died. While this can provide some basic information about the physical aspects of the organ, a non-functioning brain doesn’t give away many of its secrets.
Thanks to rapid improvements in modern technology, scientists and clinicians now have access to a range of neuroimaging techniques and neuroscience methods, such as electroencephalogram (EEG) and transcranial magnetic stimulation, that can provide a window into the living, conscious brain. These are powerful tools that can be divided into two main categories:
- Structural methods that can locate problems within the brain such as tumours or blocked blood vessels.
- Functional methods which allow researchers to study and analyse brain function and to watch how the brain reacts to different situations in ‘real time’, useful for diagnosing metabolic diseases such as Alzheimer’s disease and for cognitive and neurological psychology research.
These breakthroughs have allowed us to learn more about the brain in the last 20 years than in all previous centuries combined.
Conclusion
The human brain continues to astound and exasperate us. We have studied, labelled and dissected it, but for every question we answer, a hundred more crop up. We are only now beginning to understand the vast complexity, ability, capacity and potential of this incredible organ. When it comes to the brain, the more we know, the more we begin to realise just how much is still unknown, and how far we are from a complete understanding of the brain and how it works.
While our knowledge of the brain has more than doubled in the last 20 years, we are still decades away from discovering all its secrets. It may be that we will never know them all. With brain technology continuing to develop at such a rapid pace, who knows what we will discover next? There’s no doubt that, whatever it is, it will amaze and perplex us all over again.