Exploring the unknown with the Square Kilometre Array
Back in the early 1990s astronomers asked themselves what sort of telescope would be needed to investigate the astronomical questions of the new millennium. The answer: a radio telescope with a total collecting area of one square kilometre and 50 times the sensitivity of any existing telescope. From this came the concept of the Square Kilometre Array (SKA), a project that has drawn together scientists and governments from 20 different countries, and when completed in 2030, will be the world’s biggest radio telescope.
Described as ‘one of the most revolutionary scientific instruments we’ve built in a very long time’, the SKA will be made up of hundreds of dishes and millions of antennas linked by around 80,000 kilometres of fibre optic cables that will feed information into what will most likely be the world’s largest supercomputer. The telescope will see galaxies like our Milky Way half way to the edge of the known universe and will look back in time several billions of years to uncover the origin of the very first stars.
What is a radio telescope?
Radio telescopes collect radio waves emitted by objects in space and convert them into pictures astronomers can use to study our Universe. Radio waves, like the light waves gathered by optical telescopes, are part of the electromagnetic spectrum.
Radio emissions have a longer wavelength than visible light so the telescopes need to be very large to produce sharp images of astronomical objects. A radio telescope uses a large dish (or reflector) to collect the radio waves, and the larger the collecting area (dish) of a telescope, the more sensitive it is—the better it is at detecting faint signals from objects that are a long, long way away. After reaching the dish, radio waves are reflected onto a receiver. The receiver detects and amplifies the signals which are processed and stored in a computer ready for analysis.
Two or more dishes (antennas) can be linked together to form a telescope known as an interferometer. The signals from each antenna can be electronically combined to imitate a single dish equal in size to the distance between them—the greater the separation, the sharper the image produced. Images of the very highest resolution are produced by connecting telescopes across continental scales around the globe, a technique known as Very Long Baseline Interferometry.
Optical telescopes must be located well away from the lights of busy cities. Similarly, radio telescopes need areas of ‘radio-quiet’ as they are highly susceptible to interference from things like television, mobile phones and industrial machinery. To reduce this interference, radio telescopes are often sited in remote and sparsely populated locations.
Australia’s radio telescopes
Australia has been a pioneer of radio telescopes since their early days. The first radio galaxies were discovered from a cliff-top at Dover Heights in Sydney using an antenna no more complicated than a television aerial. Australia has two long-established radio telescopes used by astronomers around the world for their cutting edge research:
- CSIRO’s Australia Telescope Compact Array, near Narrabri, northern New South Wales. This telescope was constructed in 1988, and has six telescopes with dishes 22 metres in diameter
- ‘The Dish’—the 64-metre radio telescope at CSIRO’s Parkes Observatory in New South Wales. This telescope has been operating since 1961, and is most famous for the role it played in helping receive the video footage of the Apollo 11 crew walking on the Moon
By comparison, the largest radio telescope in the world has a dish size of 500 metres. This belongs to the Five-hundred metre Aperture Spherical Telescope (FAST) in China, eclipsing the previous record holder which was the 305 metre dish at the Arecibo Observatory in Puerto Rico.
A rock-star of telescopes
As impressive and useful as our existing radio telescopes are, the SKA will dwarf them all. Engineers and scientists from around the world are currently working on the final design plans, but the telescope will consist of hundreds of dishes, along with millions of dipole GLOSSARY dipolean aerial consisting of a horizontal metal rod with a connecting wire at its centre antennas all working in concert to provide extraordinarily in-depth coverage of space. It will produce so much data that a huge part of the technological effort of building the SKA will go into making the supercomputers that will connect the instruments and crunch all the data.
The SKA will enable us to look into all the dark corners of the universe, and if there’s anything, or anyone, out there capable of emitting a signal as strong as the ones our airport traffic control towers create, the SKA will see it.
The telescope will be built in two separate sites, one in the Western Australia outback, and the other in the wilderness of South Africa, both far away from the radio-interference of urban areas. Precursor instruments have already been built in each location, and these instruments are already among the most powerful telescopes in existence. So, while the SKA itself is not yet here, we are already exploring the universe in ways we could previously only dream of.
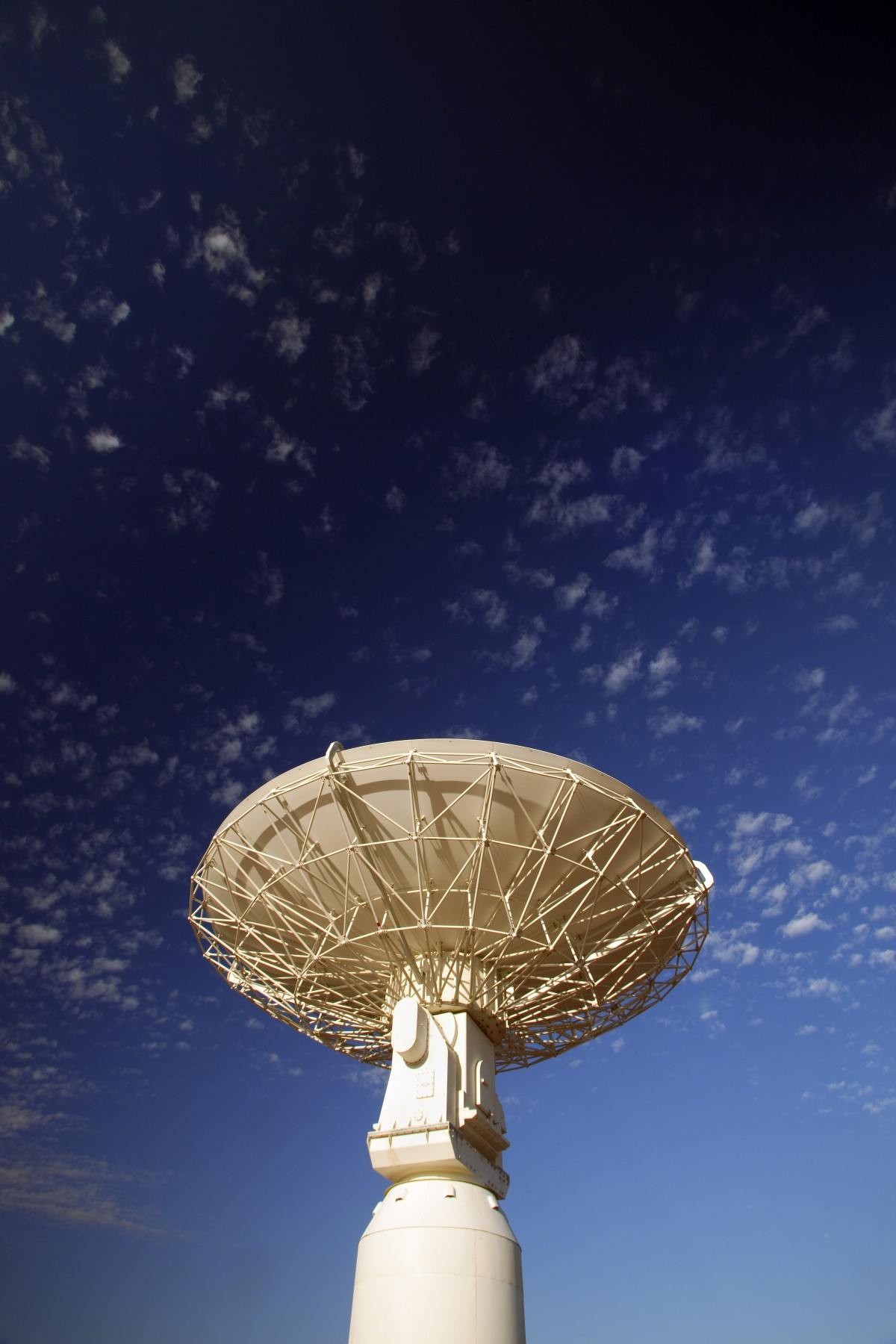
One of the 36 antennas that form the currently operating Australian SKA Pathfinder facility. Image credit: WA Department of Commerce.
Australia
SKA-LOW
The Australian site for the SKA is CSIRO’s Murchison Radio-astronomy Observatory in Western Australia, which is already home to some significant precursor technology for the SKA, the Murchison Widefield Array and the Australian Square Kilometre Array Pathfinder. These precursor instruments are enabling researchers and engineers to test out the new technologies and build upon the knowledge base that will be required to see the SKA through to completion, and are also extremely impressive instruments in their own right.
Murchison Widefield Array (MWA)
Most of the 128 dipole antenna tiles that make up the MWA are clustered together over an area of around 1.5 kilometres, with a few scattered further afield to provide better angular resolution to capture solar burst events. The MWA operates in the low-frequency range (80–300 megahertz (MHz)). It is used to search for hydrogen gas out in distant galaxies, to obtain a deeper understanding of our own Milky Way galaxy, to examine pulsing and exploding stars and to study the connections between the Sun, space weather and Earth’s environment.
Australian Square Kilometre Array Pathfinder (ASKAP)
ASKAP consists of 36 12-metre dishes that work together to form a telescope with an effective diameter of 6 kilometres. Each antenna also has a special type of wide-angle ‘radio camera’—known as a phased array feed (PAF)—which allows the telescope to obtain images of an area of the sky 30 times larger than a conventional radio receiver. The information that ASKAP gathers will be used to study galaxy formation and evolution, as well as dark energy and cosmology.
Dipole antennas
For the SKA, this site will also host around a million low frequency aperture dipole antennas. These will collect radio signals at the lower end of the radio spectrum. Some of these waves originated from a fog of hydrogen just after the Big Bang and have become stretched out as they travelled through the expanding universe. Measuring these signals will enable researchers to probe the farthest reaches of the universe and look back to the formation of the first stars, galaxies and black holes.
South Africa
SKA-MID
The South African components of the SKA will be located in the Karoo region of the Northern Cape province of South Africa. Several other African countries will also host some parts of the final SKA. At the Karoo site, several hundred mid-frequency (350 MHz–14GHz) dishes will work alongside mid-frequency aperture arrays, which are yet to be designed.
MeerKAT
Currently being built is the MeerKAT array, an important precursor to the SKA. This array will have 64 dishes, mostly contained within an area 1 kilometre in diameter. The MeerKAT gets its name from ‘meer’, the Afrikaans word for ‘more’, and the KAT-7—the Karoo Array telescope, which is an array of 7 dishes that were a precursor to the MeerKAT. The Karoo region is also home to the adorable meerkat mammal (Suricata suricatta).
Mid-frequency dishes
The MeerKAT array will be complemented by another 130 mid-frequency dishes in the first phase of the SKA project. These mid-frequency dishes, and those located at the other African sites will provide insights into how pulsars work, and probe the fundamental nature of gravity.
Although not directly connected, the low-frequency dipole array in Australia and the mid-frequency dishes in South Africa will work in concert to provide a continuous coverage over the combined range of their frequencies. This complementary nature of the instruments is what makes the SKA so powerful.
What will the SKA discover?
Twentieth-century astronomers discovered an expanding universe with billions of galaxies, each filled with billions of stars, along with exotic objects such as black holes, quasars and neutron stars. A major challenge for the next century is to understand how it all got there and how it has evolved. To this end, astronomers have identified the following key science goals for the SKA.
The birth and evolution of galaxies
When astronomers study distant galaxies, they are looking back in time. Because of the time taken for light or radio signals from distant galaxies to reach us across such enormous distances, they are observed as they were billions of years ago. The extraordinary sensitivity of the SKA means that we will be able to look much further back in time, back to a time shortly after the Big Bang when the first stars and galaxies were formed.
Many of the secrets of galaxy formation will be revealed by studying the radio emissions of hydrogen. In the early universe, hydrogen emitted radiation at a wavelength of 21 centimetres. As light from the very first stars ionised the hydrogen around them, this radiation stopped. Using spectroscopy to observe the hydrogen radiation at different times, the SKA will help us understand how the first galaxies formed and evolved over time.
Dark energy
In the late 1990s astronomers made an astonishing discovery. It has always been assumed that the gravitational attraction between galaxies would cause the expansion of the universe to gradually slow down. Two groups, one led by Australian astronomer Brian Schmidt, the other by American Saul Perlmutter, both showed that the opposite is occurring—the expansion of the universe is actually accelerating. These two astronomers, along with Adam Reiss, a member of Brian Schmidt’s group, shared the 2011 Nobel Prize in Physics.
Astronomers now believe that all the normal matter we see in stars and galaxies makes up only a small part of the total mass and energy of the universe. Most of it consists of a mysterious mix of dark matter and dark energy. The latter is driving the expansion of the universe. A fundamental challenge will be to shed light on the properties of this unseen matter-energy and to determine whether our laws of physics need a revamp. The SKA will help by conducting measurements of a billion galaxies in the universe to clarify the nature of both dark energy and dark matter.
The magnetic universe
An important discovery last century was that stars and galaxies have magnetic fields which play a vital role in controlling how they form and evolve. Despite their importance, we do not understand how the fields are created or how they maintain their strength over cosmic timescales.
By measuring subtle changes in the radio emissions from millions of distant galaxies, the SKA will be able to map the magnetic fields within the Milky Way and beyond in the vast reaches of intergalactic space. The SKA will reveal what these magnetic fields look like, their origin, and their role in the evolving universe.
Life in the universe
The SKA may answer a fundamental question as old as humankind: ‘Are we alone?’. In recent years the number of planets discovered orbiting nearby stars has grown dramatically, though it is unknown whether any harbour life.
Since the 1960s astronomers have carried out numerous searches for extraterrestrial intelligence, hoping to detect signals deliberately beamed in our direction by an advanced civilisation. The SKA will be so powerful it will be able to eavesdrop on other Earth-like planets looking for signals as weak as airport radars. The sensitivity of the SKA will also allow scientists to search for planetary systems in the process of forming and for evidence of complex organic molecules, the building blocks of life.
Testing Einstein’s gravity
Einstein’s theory of general relativity and gravity has been tested many times by astronomers and come through with flying colours on every occasion.
A major goal of the SKA will be to carry out more rigorous tests of the theory. The ideal system will be a pulsar orbiting in the ultra-intense gravitational field around a black hole. The behaviour of the pulsar will decide whether Einstein’s theory needs to be replaced by a new and more powerful theory of gravity.
Into the unknown
Although the SKA has these well-defined science goals, it is possible that its most important discoveries will be unexpected—completely new phenomena and new laws of physics.
The universe is big. The SKA will enable us to see right to its edges. Who can tell what we might find there.
This article was written by communications officers at the Australian Academy of Science, and was reviewed by the following experts: Professor Lisa Harvey-Smith, Astrophysicist, the Australian Government’s Women in STEM Ambassador and a Professor of Practice in Science Communication at UNSW; and Professor Alan Duffy, Astronomer and Director of Swinburne’s Space Technology and Industry Institute.