Do we have neighbours beyond Earth?
Expert reviewers
Essentials
- We are yet to find another planet or moon that has all the conditions needed to support life as we know it.
- There are a number of contenders for places in our solar system that might possibly harbour some sort of life: Mars, and the Galilean moons of Jupiter (Io, Europa, Ganymede, Callisto) and two of Saturn’s moons (Titan, Enceladus).
- Liquid water is the primary condition for life on other planets or moons, along with some sort of geological activity.
We have always been fascinated by the thought of alien life out there in the universe—the notion has provided the basis for a huge wealth of science fiction stories which have been limited only by our imaginations. But is there actually anybody out there? Can other creatures exist in the vast reaches of space or on other planets or moons?
The Fermi Paradox
Named for the physicist Enrico Fermi, who first outlined the argument back in 1950, the Fermi Paradox points out the strangeness of our not having come across any extraterrestrial life. Our sun is just one star among billions in our galaxy. Assuming that we’re not actually miraculously special, it seems probable that there are other planets out there like Earth that are also capable of intelligent life. This begs the question: where are all the aliens?
The Drake equation
Back in 1961, astronomer Francis Drake tried to rationalise this question by developing an equation that takes into account all the factors relevant to finding alien civilisations, and gives an estimate of the number of civilisations out there in the galaxy that should be able to communicate with us.
Several of the numbers we plug into the equation are more conjecture than solid fact, so the answer we get is not exactly definite nor exact. Rather, the equation is more of a tool for engaging interest and sparking dialogue about the issue.
Estimate the odds
Set your own estimates for each element of the Drake equation below, and see what that would mean for the number of civilisations out there in the galaxy that we might be able to hear from afar.
The search for extraterrestrial intelligence
Scientists and radio-astronomers have started the search for extraterrestrial intelligence (SETI) in a systematic manner. Several international organisations, including the SETI Institute and the SETI League, are using radio telescopes to detect signals that might have been produced by intelligent life.
They are looking for a signal of electromagnetic radiation, which travels at the speed of light in the vacuum of space. Because Earth’s atmosphere is mostly transparent in the microwave-to-radio region of the electromagnetic spectrum, SETI searches are generally conducted using radio telescopes.
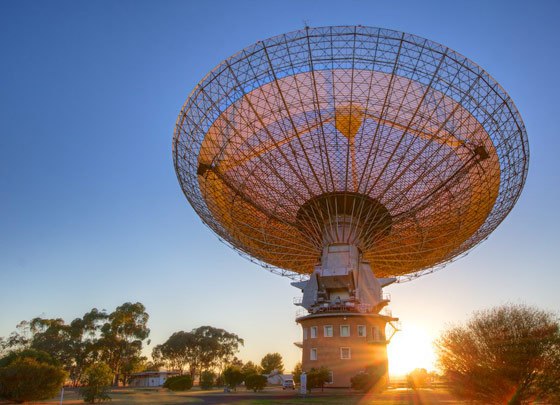
In 1995 the SETI Institute started Project Phoenix, which used three of the most powerful radio telescopes in the world: the Green Bank radio telescope in West Virginia, USA, the Arecibo telescope in Puerto Rico and the Parkes radio telescope in NSW. During its initial phase, Project Phoenix used the Parkes telescope to search for signals coming from 202 Sun-like stars as distant as 155 light years. By the end of its operations, Project Phoenix had scoured a total of 800 nearby (up to 240 light years away) stars for signs of life. The project detected some cosmic noises, but none that could be attributed to aliens.
Now anyone can become involved in the search for extraterrestrial intelligence through their personal computer. SETI@home is a project run through the University of California that makes use of the general public’s computers to increase the amount of radio telescope data that can be analysed.
While there’s currently excitement about sending human crews to Mars, missions beyond the Red Planet are at this stage pretty much unfeasible (although NASA is making noises about sending people to the icy moons around Jupiter!). The distances and travel times involved are simply too great. Basically all exploration for life beyond Earth will need to be done using robotic space probes and landing rovers. These instruments can provide a huge wealth of information and are capable of exploring as far away as Pluto, perhaps even beyond our solar system. But as for life beyond the solar system, the nearest stars are several light years away, and even communications by electromagnetic waves (which all travel at the speed of light) are essentially going to be a one-way message.
In searching for life beyond Earth, we need to think about the conditions that are needed in order to support life, to nurture those early microbes and see them evolve. The best (actually, only!) way for us to do that is to look at how life evolved here on Earth.
The origins of life on Earth
Everything we know about life comes from a sample size of one: life here on Earth. And the fact is, we don’t really know exactly how life actually did spontaneously arise from inorganic matter all those years ago … and who’s to say that different processes might not have taken place on different planets out there? So perhaps there are more questions to ask, more possibilities to consider. But let’s start with what we know.
Cyanobacteria: the simple things of life
Cyanobacteria, also known as blue-green algae, started out on Earth quite a while ago. Their fossils have been found in rocks that are around 3,500 million years old.
Although commonly referred to as blue-green algae, cyanobacteria are not actually algae. Cyanobacteria, and bacteria in general, are prokaryotic life forms. This simply means that their cells do not have distinct nuclei—their genetic material mixes in with the rest of the cell. This characteristic is distinctive of bacteria and archaea; all other life forms on Earth, including algae, consist of eukaryotic cells in which the genetic material is contained inside a membrane.
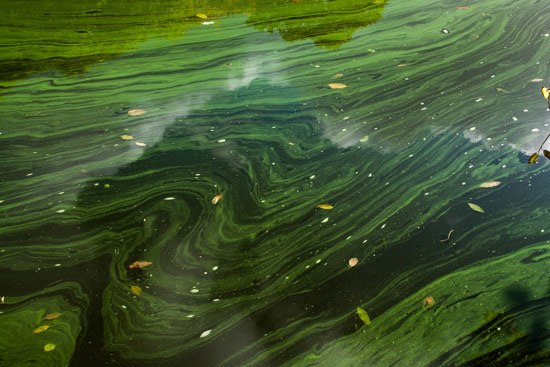
Bacteria (and archaea) are hardy creatures. They can survive in hot, cold, salty, acidic and alkaline environments in which eukaryotes would perish. Despite this, they have a bad image: after all, bacteria cause many diseases in humans, some of them fatal.
Yet without them we may not be here at all. Prokaryotes were the earliest life forms, simple creatures that fed on carbon compounds that were accumulating in Earth’s early oceans. In the harsh conditions that were present then, no other organism could have survived.
Slowly, other organisms evolved that used the Sun’s energy to manufacture their own food. Cyanobacteria then went a step further: they started to extract hydrogen from water during photosynthesis, releasing oxygen as a by-product. Over time, enough oxygen accumulated in the Earth’s atmosphere to allow the evolution of oxygen-breathing organisms.
But we may owe bacteria more than the air we breathe. It is possible that eukaryotic cells, of which humans are made, evolved from bacteria about two billion years ago. One theory is that eukaryotic cells evolved via a symbiotic relationship between two independent prokaryotic bacteria. A single bacterium was engulfed by another one, and the smaller cell continued to exist inside the other, which was beneficial to both. They evolved to become the more advanced eukaryotic cell, with its membrane-enclosed nucleus.
Regardless of how it happened, the evolution of eukaryotic cells was a significant milestone in the history of life on Earth. As conditions became more favourable, ever more complex organisms began to evolve.
Some of the oldest evidence of life on Earth is 3.49-billion-year-old fossilised remains of microbial mat structures, which look like wrinkle marks in rocks, found in the Pilbara region of Western Australia. Also found in the Pilbara region are fossilised remains of stromatolites. These are also mat-like structures of microbes that live in shallow marine environments and are still around today. Sand accumulates on top of the microbial mats, and the microbes move up towards the surface to get to the light again, making distinctive bulbous-shaped layers that eventually solidify into rocks.
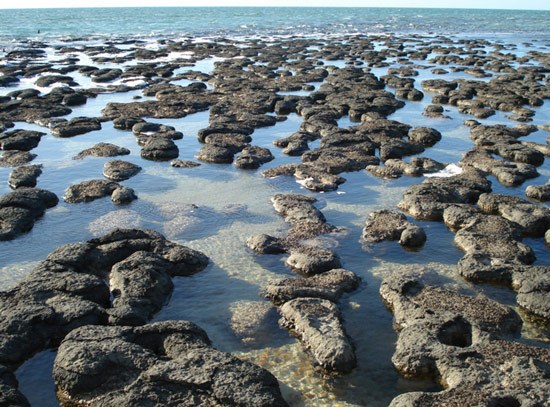
Scientists have also recently discovered 4.1-billion-year-old zircon crystals in rocks also from the Pilbara that contain specks of graphite, a carbon mineral. The graphite has a carbon isotopic signature consistent with that of organic carbon. Although this is not conclusive evidence, it has given rise to speculation that life existed on Earth even earlier than previously thought.
When we go out into the universe searching for life beyond our home planet, we think we’re most likely to find it lurking somewhere where there’s water, and where there’s a heat source to make the water warm. This seems to be the most basic criteria for an environment that can support some kind of life.
Martians?
Although Mars is literally worlds away from Earth in terms of living conditions, it is actually the most similar to Earth out of all the planets in our solar system. So it’s seen as a pretty likely contender for harbouring some sort of life form.
Mars is cold, though. The average temperature at the surface of the planet is around –63°C at the equator and gets as low as –140°C. Although living things can survive on Earth under such extreme conditions, life as we know it is unlikely to thrive at these temperatures, because the chemical reactions that sustain it would slow down substantially.
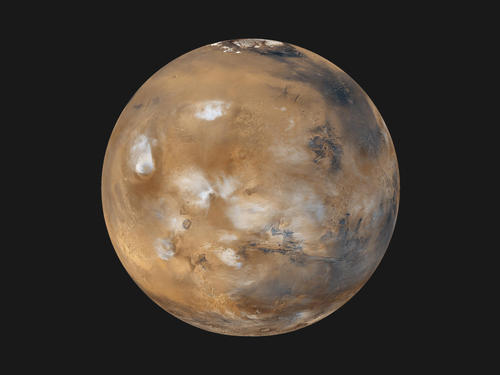
However, Mars may once have been much warmer than it is today. Scientists have detected evidence that water and carbon dioxide have escaped from the planet into space. If these gases were once present in the planet’s atmosphere in large enough quantities, they could have created a ‘greenhouse’ effect similar to the one which keeps Earth warm. It’s thought that around four billion years ago the conditions on Mars might have been wetter and hotter, and probably therefore more conducive to life.
In August 1996, there was a lot of excitement, and then disappointment, when scientists from the National Aeronautics and Space Administration (NASA) announced they had detected possible signs of life on Mars in a meteorite found in Antarctica. The meteorite, called ALH84001, contained microscopic chain structures that could be interpreted as bacteria-like forms.
These included carbonate rosettes—tiny blobs, similar to rosettes produced by bacteria in ponds on Earth as they metabolise minerals. The meteorite also contained an unusual mixture of chemical compounds known as polycyclic aromatic hydrocarbons (PAHs), which might have been produced as once-living organisms decomposed. There were also tiny crystals of magnetite and iron sulphide embedded in places where the carbonate rosettes had dissolved. Some bacteria on Earth also manufacture similar crystals.
Heated discussion ensued, and it was eventually concluded that these were most likely not the fossilised remains of microbes and microbial activity, and that geological processes, and contamination, could account for all the observed ‘evidence’. So much for the Martian microbes.
Methane
The debate about life on Mars continued when methane was detected in the Martian atmosphere in 2003. The Curiosity rover has also now found methane, after not finding it in early experiments. This evidence is not conclusive, as although methane can be produced biologically, volcanic and geothermal processes also contribute to methane emissions.
Water
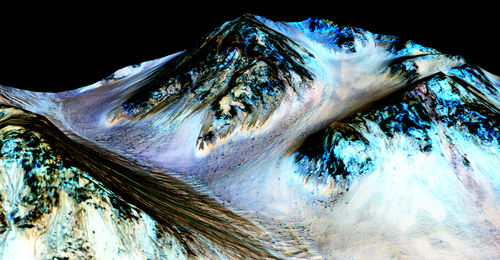
Water is crucial for life on Earth. Although Mars has an abundance of geological structures that look like they could only have formed in the presence of running water, there’s not much of it there now. There’s frozen water at the poles, and also frozen water in the soils, and scientists have recently found evidence for some flowing liquid water, in the form of ‘recurring slope lineae’. These are flows of running salty water that are seen as streaky lines on slopes in a few locations on Mars’s surface. Hydrated salt crystals (salts with water bound up in their crystal structure) have also been found near the streaky formations. The water is full of perchlorate salts, which help to lower its freezing point, enabling it to be liquid in the cold conditions of Mars.
If there ever was life on Mars, it should still be there, somewhere, and NASA’s next Martian mission, the 2020 rover, is going to continue to look for it. The pressing question at the moment is: where exactly should it look? The engineers want to send the rover to an easy, safe landing site … but the most likely place to find life would be far more adventurous, near volcanoes or sites that may have once been hot springs. Or perhaps near the recurring slope lineae—the intriguing flows of liquid water that could possibly be home to some sort of microbes.
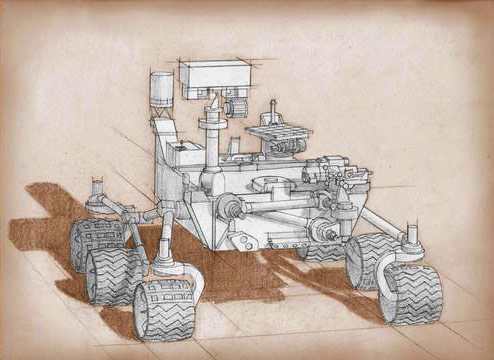
The Galilean moons of Jupiter
Although some have speculated that life may be possible within the (unhospitable to us, with its extremely high gravity, unpleasant gases and high magnetic field) atmosphere of Jupiter itself, more likely candidates are the four icy Galilean moons. All four moons are extremely cold, and all have thin atmospheres. Europa’s and Ganymede’s atmospheres are so thin they’re known as exospheres (so thin that the molecules drift around without ever bumping into each other) while the atmospheres on Io and Callisto are known as ‘collisional’, which means the atmosphere is dense enough to sustain wind and weather.
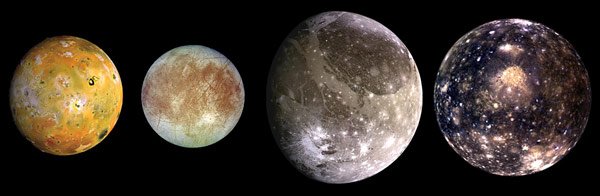
Io
Io is the closest moon to Jupiter, and a veritable hotspot of volcanic activity. Rather than internal convection, which is responsible for the volcano-tectonic activity we have on Earth, Io’s dynamism comes from something called ‘tidal flexing’. Gravitation pulls from Jupiter’s next two moons, Europa and Ganymede, have tugged Io’s orbit into an oval shape. As Io orbits around Jupiter, its oval-shaped orbit means that Jupiter’s extremely strong gravitational pull is stronger at some times during the orbital path, and weaker at others. The uneven gravitational pull causes the moon to bulge, then bounce back, causing friction inside Io’s interior, driving its intense volcanic activity. Io is the most volcanically active moon in our solar system, with plumes of material reaching up to 300 kilometres from the surface, spewing out masses of what is possibly either silicate rock or sulfur-rich material into space.
The upshot of this is that because Io is a dynamic, active moon it gets one fairly big tick for being possibly able to support life. However, for all its tidal heating, the surface of Io is around –143 °C. And although Io’s atmosphere is one of the thickest of all the moons in the solar system, it’s still extremely thin—Earth’s atmosphere is around 200 million times denser. The other thumbs down for Io is that it is bathed in a huge amount of radiation from Jupiter. There’s also no evidence of any water on the moon. However, scientists believe that water may have been present on Io early in its formation, and there is a possibility that some form of life may exist underground.
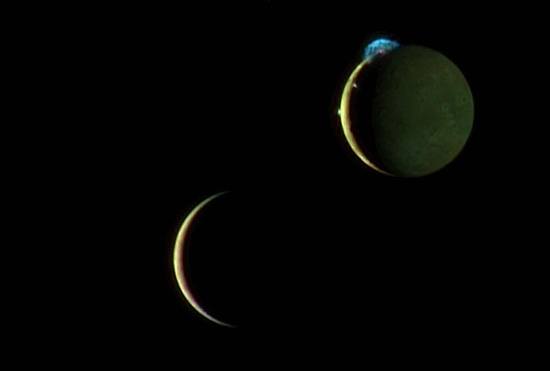
Europa
Mars has ice caps, frozen soils and some dribbles of salty water that have got people very excited. Underneath an icy crust, Europa is thought to have a huge, planet-wide liquid water ocean. All life on Earth requires liquid water, and so what better place to look than an ocean? Furthermore, Europa’s ocean would be protected from Jupiter’s radiation, and rather warm. It would be not much below freezing temperature, compared with the chilly –160 °C on the surface.
NASA’s Galileo mission returned lots of images of Europa’s surface that clearly show some extensional features and ridges on the icy crust, suggesting that convection is occurring beneath it. This sort of activity is a plus for life. It was also the Galileo mission that noticed how Europa seems to alter Jupiter’s magnetic field as it moves through it, which is the best evidence we have yet of a salty ocean under the moon’s icy crust.
The Hubble Space Telescope has also taken snaps of something that could be a geyser of water, erupting around 200 kilometres above the surface of Europa. Europa’s gravity is quite strong for an icy moon, meaning that the water is pulled back down to the surface of the moon, where it freezes.
All in all, Europa is somewhat of a favourite in terms of the potential for finding life beyond Earth. NASA is planning its Europa Mission, which will place a spacecraft in orbit around the moon, equipped with cameras, spectrometers, an ice-penetrating radar and a magnetometer to snatch data from Europa during the 45 fly-bys planned.
What’s more, NASA is thinking about building a rover that could potentially explore the ocean of Europa. It would dig/drill through the icy crust, and travel with its wheels riding along the underneath of the ice, essentially floating up against the underneath surface of the ice.
Ganymede
Ganymede is Jupiter’s largest moon, made of roughly equal amounts of rock and ice. Other than a very weak atomic oxygen atmosphere, there’s no breathable air. Ganymede is pretty special—it’s the only moon in our solar system to have its very own magnetic field, probably caused by convection in its iron-rich liquid core. It also has a very thin atomic oxygen atmosphere, formed by splitting of water molecules when ice sublimates.
The magnetic field causes auroras, which are affected by Jupiter’s magnetic field. Using the Hubble Telescope, scientists studying how those auroras changed (or rather, didn’t change as much as expected) with shifts in Jupiter’s magnetic field surmised that Ganymede has an ocean of salty water beneath its icy surface. Ganymede’s auroras are ‘rocked’ by changes in Jupiter’s magnetic field. However, they don’t appear to rock as much as they should, and so scientists have postulated that it is the presence of a salty ocean which creates a second magnetic field that counteracts Jupiter’s. This creates ‘magnetic friction’ that suppresses the rocking of Ganymede’s auroras.
The ocean is thought to be around 100 kilometres deep, beneath around 150 kilometres of ice on Ganymede’s surface. A 100-kilometre-deep ocean of salty water that is possibly full of Ganymedean oceanic critters?
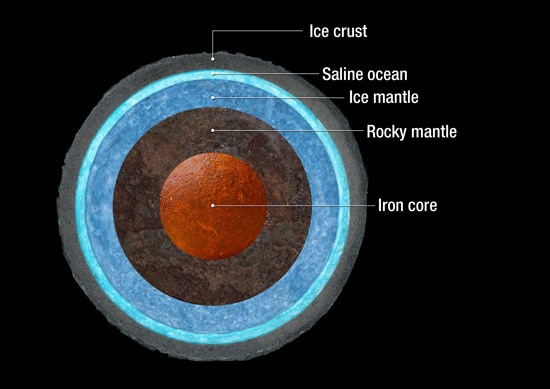
Another theory is that Ganymede’s interior is made from layers of salty liquid ocean interspersed with layers of ice, which result from the way salty water and ice behave under extreme pressure. Salty, dense water will sink to the bottom. At extreme pressures, it gets even more complicated. Even though the ice we’re used to will float on water, at very high pressures the ice becomes more compacted and more dense. However, when ice forms, the salts don’t freeze with it. They precipitate out into the surrounding water, making it even more dense—so much so that it sinks down below even the very dense ice.
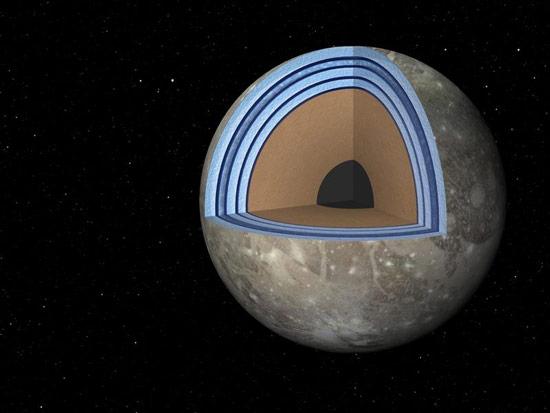
The European Space Agency’s JUICE mission hopes to check out Jupiter and the Galilean moons in more detail in the 2030s.
Callisto
Of the Galilean moons, Callisto is the furthest from Jupiter. Callisto has a very thin atmosphere, is thought to contain an ocean, and is therefore another possible contender for life beyond Earth. However, its distance from Jupiter means it doesn’t experience such a strong gravitational pull, so it’s not as geologically active as the other Galilean moons of Io and Europa. On the flip side, this also means that it’s sufficiently far away to not be blasted by (generally life-unfriendly) radiation from Jupiter.
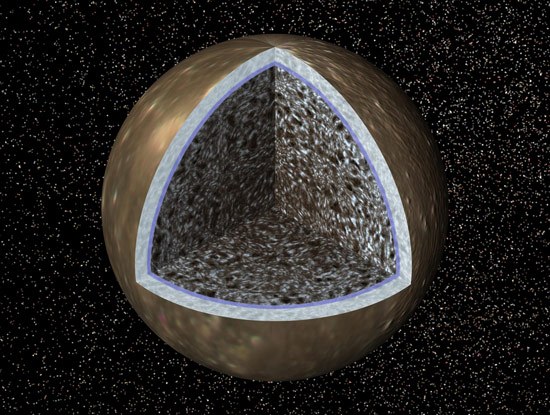
Saturn’s moons
Titan
Titan is Saturn’s largest moon, and it’s a remarkable place. It has a thick atmosphere—even thicker than Earth’s. Its atmosphere is primarily composed of nitrogen and methane, and it’s chock-full of organic compounds—made when sunlight breaks down methane—which are thought to rain down upon the surface.
Titan is cold, with a surface temperature of around –180 °C. There are rivers and lakes on the moon’s surface. They’re not filled with water, though. They are thought to contain an oily mixture of methane and another hydrocarbon called ethane. Going from our Earthly evidence we tend to think that water is an essential ingredient for life. But, really, all life needs is liquid in which the necessary chemicals can dissolve and interact with each other. What’s to say that that liquid can’t be a hydrocarbon? DNA, the blueprint for all life on Earth, isn’t soluble in methane, but perhaps a potential life form on Titan could be based on some other sort of chemical structure. Or perhaps Titan can be examined in the context of a ‘proto-biotic’ world, where we could find clues to how life evolved on our own planet … it’s certainly intriguing.
NASA is thinking about building a submarine capable of exploring Titan’s murky hydrocarbon depths.
Enceladus
Encleadus is another cold, frozen moon, though much smaller than the others described above. It’s only around 500 kilometres in diameter. Like Europa, Ganymede and Callisto, it’s thought to contain an underground (well, under-ice) ocean. Beneath a 30–40 kilometre-thick icy crust, the ocean is around 10 kilometres deep. Cracks and extensional features within the icy crust provide evidence that the moon is active. The activity is also driven by tidal flexing, this time under the pull of Saturn’s gravity.
Also, like Europa, Enceladus has geysers of water erupting from its surface. Being much smaller in size than Europa means it has weaker gravity, and at least some of the water from Enceladus’ geyser escapes to space.
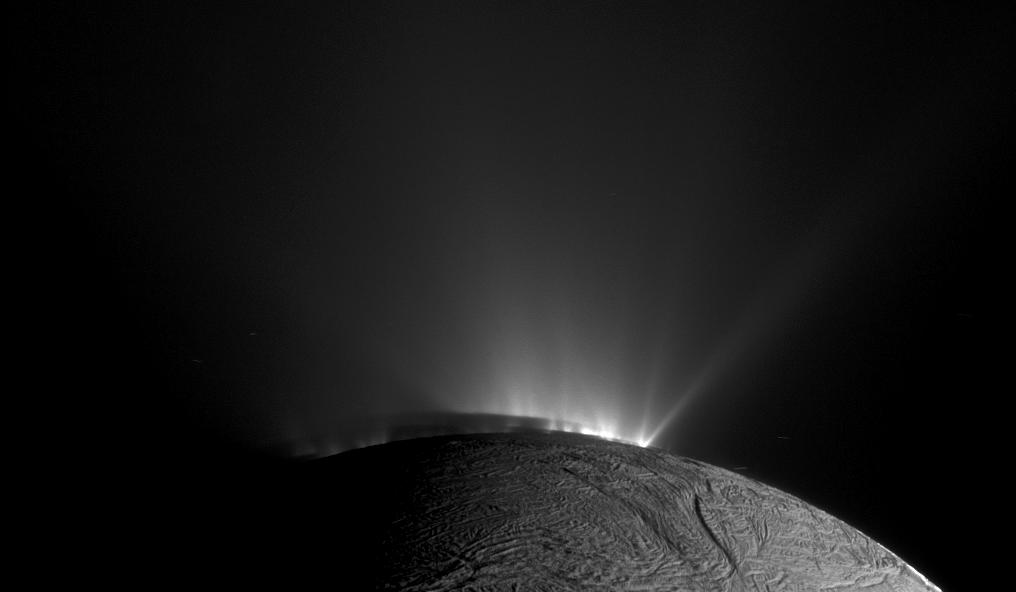
Life? Well, the water spouting from Enceladus’ south pole geysers has been sampled by the Cassini space probe, and has been found to be salty and contain organic molecules, the basic ingredients for life. So it’s pretty promising, but there’s no direct evidence yet. At the time of writing, everyone is waiting with bated breath to hear the findings from Cassini’s deepest ‘dive’ yet into one of Enceladus’ plumes, where it passed by the surface of Enceladus at a distance of just 50 kilometres.
There’s a complex set of conditions needed to create and sustain life, and we’re yet to find somewhere that fits the bill or ticks all the boxes. Yet the prospect of ticking those boxes is tantalising: who or what is actually out there? And will it, or they, help or hinder us?
Two possibilities exist: either we are alone in the Universe or we are not. Both are equally terrifying.Arthur C. Clarke
Terrifying or not, we’ll keep on looking.