What has chemistry ever done for you?
Expert reviewers
Essentials
- Chemistry is a fundamental and enabling science that investigates molecules—the building blocks of all matter—and how they interact to affect the composition, structure and properties of substances.
- The industrial applications of chemistry directly affect our daily lives—what we eat, what we wear, our transport, the technology we use, how we treat illnesses and how we get electricity—to name just a few.
- Research is constantly deepening our understanding of chemistry, and leading to new discoveries.
- Chemistry will help us solve many future problems, including sustainable energy and food production, managing our environment, providing safe drinking water and promoting human and environmental health.
To many, chemistry is a foreign concept, belonging to the world of academia and textbooks with little relevance to our everyday life. In fact, you would be hard-pressed to find an aspect of your daily routine that isn’t directly impacted by chemistry research.
Chemistry is the study of molecules: the building blocks of matter. It is central to our existence, and leads our investigations into the human body, Earth, food, materials, energy, and anywhere and everywhere in between. The chemical industry, supported by chemistry research, underpins much of our economic progress, and provides wealth and prosperity for society. In Australia, 60,000 people are employed by the chemical industry and it contributes around $11.6 billion annually to our GDP.
What follows is a snapshot—just a small sample—of the major discoveries in chemistry that have helped to shape the way we live. From the first instances of metal work in the Copper Age in 5000 BC, to the digital era and the new cutting-edge technologies of today, such as nanoscience and biotechnology, chemists have more often than not been the driving force behind the progress in our standard of living.
Metals
Chemical theory has been developing since well before ‘chemist’ became a possible career choice. The phenomenon of fire was one of the first marvels that humankind sought to understand and the use of fire led to the study and manipulation of metals. This dates back to 5000 BC, when copper was first discovered and replaced stone as a material for making tools. It was obtained by a process called smelting GLOSSARY smeltingextracting metal from ore (a mixture of rock and metal) by heating and melting , and was thought to also have produced the first glass as a side product.
The Bronze Age arose when it was discovered that copper could be combined with tin to produce a harder metal—you guessed it, bronze. This was the first alloy GLOSSARY alloya mixture of metal with other metals or non-metals. Common alloys include steel, brass and pewter. They are often cheaper than elemental metals, with many similar or enhanced properties. ever made and resulted in stronger weapons and tools. The trading of these tools facilitated sharing of technology and knowledge between early civilisations. The Iron Age, following at around 1200 BC, saw the prevalence of iron increase as the major metal of choice for cutting tools and weapons. Iron was slower to evolve as a material because it required higher temperatures to work the metal. This shift saw a change in smelting practices, improved furnace technologies, and also the development of forging GLOSSARY forgingshaping of metal using compressive forces, such as hammering , as opposed to the casting GLOSSARY castingHot liquid metal is poured into a hollow cavity, and cooled in order to solidify it into a desired shape. techniques used in the Bronze Age.
.png)
Materials and manufacturing
The Iron Age also saw the development of many of the basic elements of urban development we are familiar with today such as cements, mortars and bitumen. During this period, populations became increasingly urbanised in large cities leading to construction of the first proper roads.
Around 500 years ago, chemistry became an earnest occupation. Elements other than naturally occurring metals were being identified and their properties were being explored—though they were still not entirely understood. People didn’t yet have much of an appreciation of the underlying science that determined material properties and it was unclear how many different basic or elemental building blocks there were.
Another important development was that of vulcanised rubber GLOSSARY vulcanised rubberhardened, more durable rubber, obtained by adding sulfur at high temperatures , in 1843 by Charles Goodyear. This led to pneumatic GLOSSARY pneumaticcontaining a cavity of air; operated by gas or air under pressure tyres and kicked off the polymer and plastics industries, which would later revolutionise the manufacture of household goods. The discovery by Alfred Nobel of dynamite in 1867 and more refined explosives later on led to rapid expansion of mining as a means to extract ores and minerals.
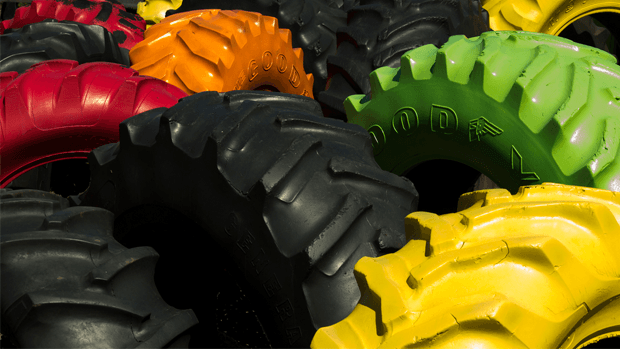
The synthesis of the first artificial dye, a purple colour later named mauveine, occurred in 1856. It was an accidental discovery made by William Perkin, an 18-year-old who was actually trying to make artificial quinine. Blue and purple pigments were historically incredibly rare, and mauveine was met with high demand. Its development drove further investigation into organic chemistry, and the production of related dyes and pigments. Some of the world’s biggest organic chemistry companies today were founded around this time due to demand for dye production.
Despite the increasing use of chemical compounds, it wasn’t until 1870 that Dimitri Mendeleev came up with a systematic way to arrange all the known chemical elements into the periodic table. The table is based on common chemical properties and trends in their behaviour. It is a concise, information-dense catalogue of all of the known different types of atoms, and it is still an essential tool for chemistry study today.
More recently, Harold Kroto, Richard Smalley, James Heath, Sean O'Brien and Robert Curl at Rice University made a new discovery regarding something we thought we thoroughly understood—they discovered a new form of carbon. Kroto, Curl and Smalley were later awarded the 1996 Nobel Prize in Chemistry for discovering fullerenes, a completely new arrangement of carbon atoms, forming ball-shaped cage-like structures. These have been useful in materials design and could have a number of biomedical applications.
This field of research has also led to the development of carbon nanotubes. Carbon nanotubes are used to create ultra-strong, lightweight materials, for use in aircraft for example.
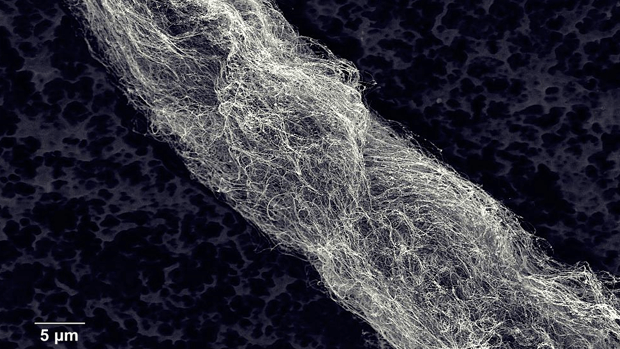
Another form of carbon that has unique properties is graphene. Graphene is a sheet made of a single layer of carbon atoms, and although a single layer of atoms may sound extremely flimsy, it’s actually extremely tough, 200 times stronger than steel, ultra-light, flexible, and an excellent conductor. Although scientists knew for quite a while that graphite was made of sheets of carbon atoms, it was only in 2004 that Professors Andre Geim and Kostya Novoselov were able to isolate a single layer on its own to make graphene. Graphene isn’t yet as ubiquitous in our everyday lives as some other great chemical discoveries—with this one, it’s more of a case of ‘What will graphene do for you in the future?’ With its unique properties, it has the potential to have a huge impact in several areas, including electronics, materials, energy technology and biomedical applications.
Energy
One of the key contributions the field of chemistry has provided to our burgeoning society is the ability to harness and store electrical energy—electricity. Electricity had long been an intellectual curiosity, and the phenomena became more understood through experiments by chemists and physicists.
Traditional energy production, via combustion and thermodynamics of fossil fuels, led to the industrial revolution. This boom in industry from the mid-1700s to the 1800s was an era of growth, with chemical engineers coming to the fore to scale up and industrialise processes of manufacture. It was during this time that many of the practical uses of chemistry we rely on today were developed.
The batteries that so many of our devices depend upon are underpinned by a chemical reaction that produces electricity. The first electrical battery was created by Alessandro Volta, who proved that electricity flowed through wires attached to different metals and the types of metal used affected the voltage. The term ‘volt’ as a measure of electric potential is named in honour of him. Although current batteries are far more sophisticated than in Volta’s day, there is renewed interest in pushing this vital chemical technology further, so that sustainable energy produced by solar cells or wind energy can be stored.
Food and agriculture
Complex technology goes into modern food production. From soil science to nutrition analysis, and from safety testing to food packaging and preservation, the chemical processes involved are extensive, and often not given much consideration. For example, if it wasn’t for refrigeration, our food distribution systems would be limited and storage would be short lived. The first cooling systems were developed in 1874. They used dimethyl ether, but it wasn’t long before ammonia-based systems were introduced, which are still in use today in industrial refrigerators.
Ammonia is also an integral chemical innovation for food production, chiefly due to its use in fertiliser production. Indeed it is estimated that around 1 per cent of the world’s energy is used to make ammonia. Increasing the productivity of our food-growing systems has become necessary due to the combined pressures of population increase, climate change and water shortages. If it wasn't for the Haber-Bosch process, our current agricultural output would be unsustainable. It was first developed in 1909, and allows for the efficient, large-scale production of ammonia (NH3) by reacting atmospheric nitrogen (N2) with hydrogen (H2) at high temperature and pressure. This resulted in an easily accessible route to fertiliser production, and was responsible for quadrupling agricultural productivity. The discovery of pesticides and herbicides further increased crop yields, with DDT and glyphosate being key compounds. Today, around 40–60 per cent of global agriculture yields rely on artificial fertilisers.
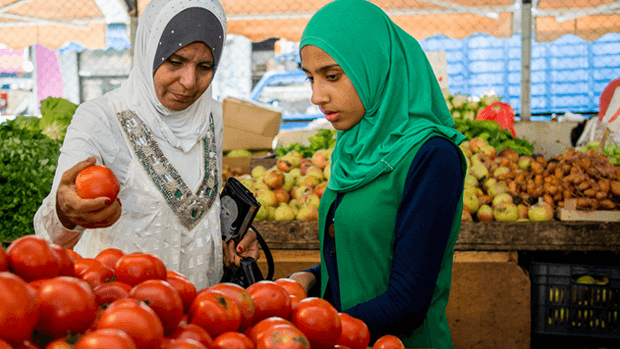
Human populations the world over rely on chemistry to maintain safe clean water supplies. Recycling will be essential for sustaining this resource into the future. Here in Australia, droughts forced us over the past decade to reduce our water use and reconsider our reliance on dams and reservoirs, and think about alternative water sources. There are already three large water desalination plants, in Sydney, Melbourne and Perth. Without this development in chemical engineering, nations including Saudi Arabia, Kuwait, the United Arab Emirates, Bahrain and Libya would most likely not have enough usable water to sustain their current populations. Effective resource management is becoming more important as we face environmental uncertainty, with chemistry playing a crucial role in potential solutions.
Health
Modern health care is founded on many life-saving breakthroughs the field of chemistry has provided. These include developing new pharmaceuticals, diagnostic tools and better diagnostic equipment such as X-ray machines, MRI imaging, cancer tests and pregnancy kits. Analytical chemistry and forensic science are crucial for identifying poisons or toxins in food, plants and animals, and in tracing and identifying unknown chemicals and materials.
Medical practices have also drastically changed as chemical knowledge has advanced. The discovery of painkillers and anaesthetics opened up a whole new scope of opportunity for medical practitioners. Advanced surgery (rather than simple amputation) became possible. Compounds such as nitrous oxide GLOSSARY nitrous oxidea sedative agent also known as laughing gas, nitrous, nitro or NOS (N2O), or laughing gas, became popular and minor surgical procedures and dental work became slightly less risky, although infection was still a major challenge. Here, chemistry came to the rescue (again!) with the first antiseptics. In 1867, Joseph Lister introduced carbolic acid as an antiseptic to clean surgery wounds. Death rates in his surgery dropped from 45.7 per cent to 15 per cent.
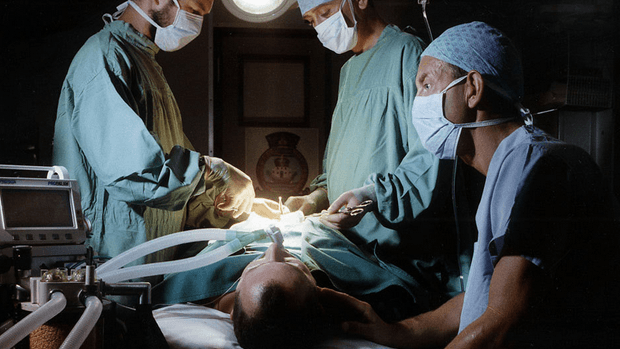
Still on this front, but somewhat later, Alexander Fleming discovered the first antibiotic, penicillin, in 1928. This discovery ushered in a whole new era in fighting bacteria-borne illness. It wasn’t until the 1940s however, when Howard Florey, a scientist from Adelaide, manufactured penicillin on a large scale that it was adopted for widespread use. His work resulted in the easy treatment of infection, and has also saved millions of lives. But the microbes have begun to fight back, meaning our days of simply solved infections may soon be behind us. Due to the ever-increasing prevalence of antimicrobial resistance, further work in this field of chemistry is more important than ever.
Marie Curie was the first woman to receive a Nobel Prize, and the first person to receive two Nobel Prizes and to this day is one of only two people to hold two in two different, scientific fields (physics and chemistry). She is an important icon for science and in particular for chemistry, as her work in the discovery of
radioactive elements
GLOSSARY
radioactive elementsunstable elements that decay with time. They have no stable naturally occurring form.
provided the base work for innovations in X-ray imaging, nuclear power and radiotherapy.
In 1953, Francis Crick and James Watson published the structure of and mechanisms of DNA, which built largely on the work of Rosalind Franklin and Maurice Wilkins. Crick, Watson and Wilkins were awarded the 1962 Nobel Prize in Medicine for this discovery, by which time Rosalind Franklin had unfortunately died from cancer. This work has since helped to explain how diseases are passed down through generations, and explains other mysteries such as why we look like our parents, how cells function on a micro level and how life evolves. It was a pivotal moment for academic research, and has shaped the direction of research into medicines and health, with a push towards personalised medicine.
Technology
One aspect of chemical innovation that's largely taken for granted but is integral to the day-to-day life of many people now are the display screens in smart phones, televisions and computers. These devices use molecules known as liquid crystals to control light and images, which gives them their name—LCD (liquid crystal display) screens. Liquid crystals occur when a substance has an intermediary state between a solid and a liquid. Instead of having one melting point, describing the change from solid to liquid, a liquid crystal has two—an initial temperature at which a substance melts to form a turbid GLOSSARY turbidcloudy, opaque, or thick with suspended matter fluid, and a clear secondary melting point at which that turbid fluid becomes clear. Between these two points is the liquid crystalline state.
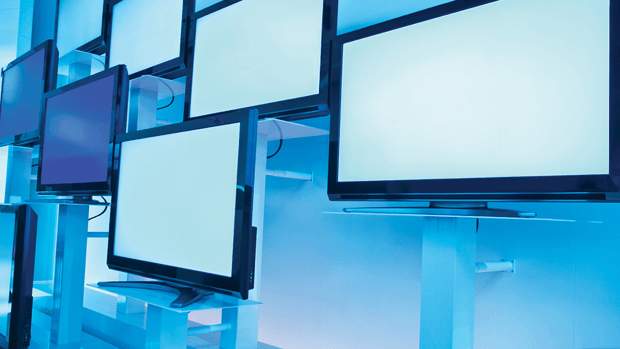
Liquid crystals have light modulating properties, and as such are useful in screens. They were first observed in 1888 by botanist and chemist Friedrich Reinitzer, who observed the effect in cholesterol extracted from carrots. LCD technology is likely to be superseded by light emitting diodes (LEDs) in the next decade. LED displays are more durable and consume less electricity. Their impact will depend on further materials breakthroughs in the fields of conducting polymers and nanomaterials such as quantum dots, which provide the bright, vivid colours needed for display screens.
Conclusion
The consequences of chemistry are far reaching. Chemistry has been largely responsible for shaping society as we know it; from developing stronger materials for large scale construction, down to what kind of cosmetic products you use every day. Society has benefited hugely from advances in the field, with the few key discoveries outlined here just a small cross-section of the chemical innovations that have driven society’s development. While discoveries in chemistry have made a huge impact, and continue to have emormous potential, we also need to ensure that we use them responsibly to ensure sustainability into the future.