Going back in time ... with mud
Expert reviewers
Essentials
- Earth’s history is recorded in the rocks that formed as the planet evolved.
- Physical characteristics of rocks give clues to how they were created and the environments in which they formed.
- Layers of mud and other sediments that accumulate at the bottom of oceans also provide links to past environments.
- Detailed climate histories can be pieced together by looking at oceanic sediments and the microfossils they contain.
Earth is old—4.9 billion years to be approximately exact. Scientists have a pretty good idea of how Earth’s climate has changed throughout the planet’s history, as it has passed through ice ages and back into warmer periods and back again, with the most detailed records extending back around half a billion years. There certainly aren’t any instrumental records that go back that far in time, so how is it possible to know what Earth was like millions of years ago?
The rock record
To figure out how our planet has changed over time, we have to look at something that has persisted throughout Earth’s lifetime: the rock record. By looking at changes in the types of rocks that we can see around the world, geologists can put together the history of the planet’s evolution. Sedimentary rocks—rocks formed from the accumulation of sediments—are particularly useful when reconstructing the environmental and climatic history of the planet.
While it’s possible to find huge sedimentary outcrops on dry land, the ultimate fate of a huge proportion of sediments is the bottom of the ocean. Small mineral and organic particles are transported to oceans by a variety of natural means, and form thick sediment blankets on the seafloor. Scientists are able to piece together the environmental history for some regions of the planet by exploring the mud at the bottom of the ocean.
Sedimentology 101
To interpret the history that can be extracted from layers of seafloor mud, you need to know the basic principles of sedimentology—the study of how modern sediments are created, transported, deposited and eventually turn into rock. The sediments at the bottom of the ocean are clastic sediments, which are particles that have been eroded and broken down from other rocks and combined with a lot of organic material—skeletons, shells and other remains from creatures that either lived in the water column of the ocean or on the seafloor.
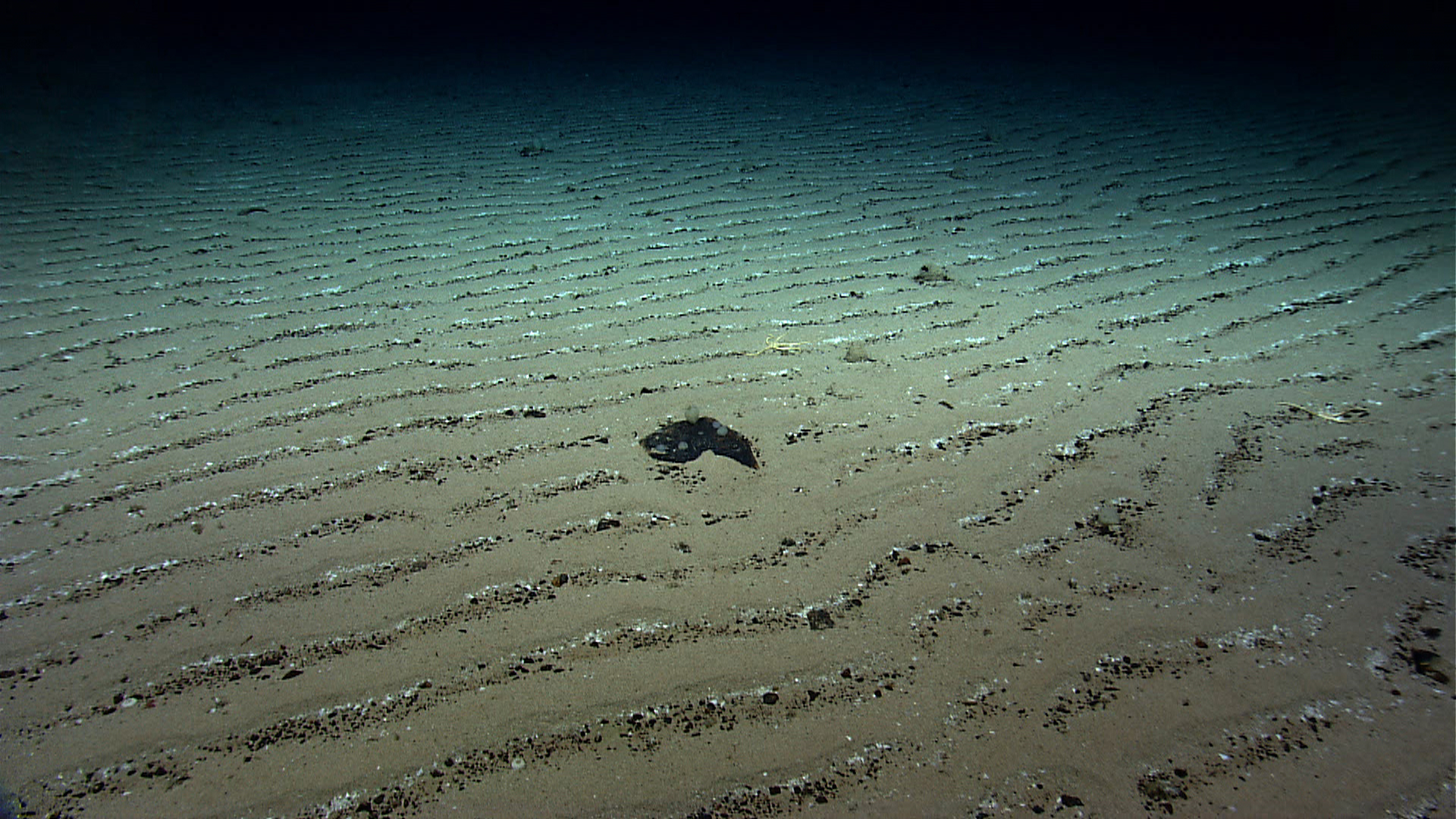
The first step in the process is the creation of sediments, where existing rocks are eroded and broken down by various processes into smaller particles. These can range in size from tiny grains of clay and sand to larger pebble-sized chunks of rocks. In this context, even large boulders can be a type of sediment.
Various processes then transport and deposit these sediments—including rivers, glaciers, landslides (which can also occur underwater) and wind. Each of these dynamic processes is different, and so the types of particles they transport and deposit can be very distinctive. By studying the features of a sedimentary rock, you can tell what sort of process originally created it.
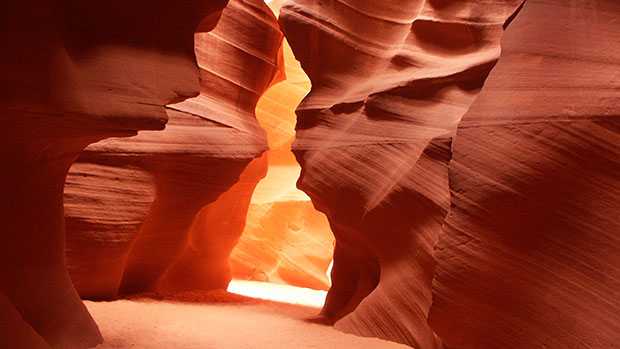
Imagine a strongly flowing river carrying lots of sediment along with it. The stronger the flow, the more particles it can transport. It’s also capable of carrying larger particles along with a range of smaller, fine-grained particles. These particles will be deposited—settle down out of the water—when the flow is no longer strong enough to carry them. Larger particles settle first, followed by smaller and smaller particles as the flow slows down even further.
Particles that have been carried a long way are usually nice and round and smooth, as all their rough edges get worn down as they are transported by the river. So you can make deductions about the distance a particle may have travelled based both on its size and its shape. Whether or not the particles are well sorted—if they’re all around the same size—or a jumble of different sizes can also provide clues on what sort of process transported them.
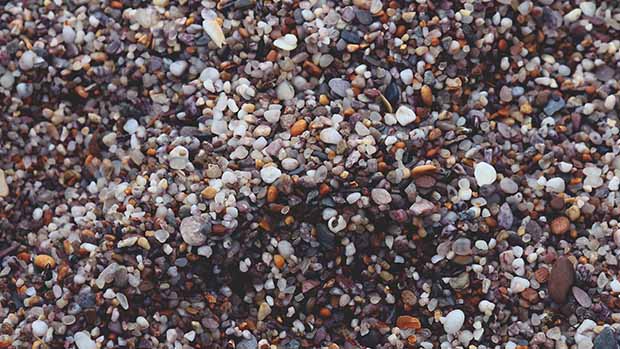
Glaciers also transport sediments, scraping them from the underlying rock and dragging them along as they slowly grind their way over the rock surface. Some sediments formed from glacial movement can be extremely fine, and are known as ‘glacial flour’. This material can stay floating in the water for a very long time, and can be easily transported by ocean currents far from land into deep sea.
Glaciers and ice sheets can also carry other bigger sediments, which are entrained in the ice flow. When icebergs containing this material break off (calve) from a glacier into the ocean, they can carry this coarser material up to hundreds (!) of kilometres out to sea as they float away. When the ice melts, the particles drop to the seabed below. This is known as ice rafted debris, and the particles can be pretty much all shapes and sizes, from sand to boulders. This is one of the only ways to carry larger material into deep quiet water where the ocean currents are too weak to deliver anything bigger than very small, fine-grained material, like silts and clays. When you see large rocks lolling about in a bed of otherwise fine sediment, it’s a pretty safe bet there were active glaciers nearby at some point in the past.
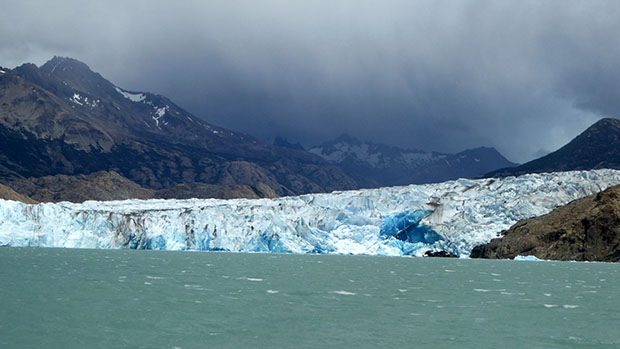
If you find a sedimentary rock with a pattern of larger sediments at the base that grade to finer sediments in the upper layers of the rock, it’s likely that it formed from some sort of a landslide or slope collapse on the sea floor—where a whole lot of sediment was transported all at once in a single chaotic event.
The same principles apply to the layers upon layers of mud and other sediments that have accumulated over thousands—even millions—of years on the seafloor. Again, the physical characteristics of the sediments provide a lot of information about the processes that led to their deposition.
Another clue to the past in sediments and sedimentary rocks is the presence of fossils. If you can see fossilised pieces of coral and seashells in your rock, you can be pretty sure that rock formed in a location that used to be under the ocean. Even more information can be gained from identifying the species of fossil present. By comparing them to modern examples of that species, you can make some educated guesses about the ancient environments that the fossils preserved in the sediments lived in.
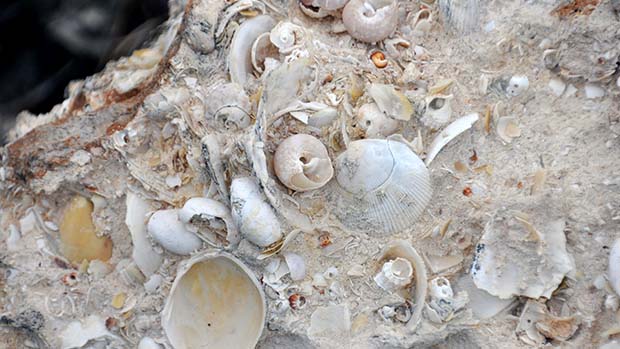
Even in the deep sea, most ocean sediments can contain microfossils—the remains of tiny organisms that lived in the water and settled down onto the seabed after their death. These include foraminifera and radiolarians, which are types of zooplankton—tiny creatures that eat other types of plankton to survive. Also common in ocean sediments are diatoms, a type of phytoplankton. These are tiny plant-like organisms that rely on photosynthesis to create their energy. Pollen spores that are also sometimes preserved in the sediment can provide information regarding the types of vegetation present on nearby land.
One of the main uses of all these microfossils is biostratigraphy—a method of figuring out the age of sediments by examining the microfossil species. As various species have evolved and become extinct over time, the presence (or absence!) of particular species can give a rough idea of the age of the sediments.
More about microfossils
Foraminifera
There are many species of foraminifera, affectionately known as ‘forams’. Forams have varying preferences for warm or cold water, high or low oxygen availability, and ability to deal with feast or famine conditions, and so just knowing the species of foram present in sediments can give a pretty good idea about the climate they lived in. If you find polar species, you know that at the time those sediments were deposited, the region wasn’t a tropical oasis—it would be like finding a penguin on a tropical island!
Additionally, they generally build their shells, called tests, from calcium carbonate (CaCO3), a mineral made from calcium (Ca), oxygen (O) and carbon (C). They pull these elements from the seawater, which means that some of the chemical characteristics of the seawater are preserved in their shells. Small changes in foram shell chemistry enable scientists to construct records of seawater temperature, or how much of Earth’s water was locked up in ice sheets in the past. Furthermore, for forams younger than around 50,000 years old, scientists are able to use carbon-dating methods to analyse the carbon in their shells and obtain a pretty precise age for the layer of sediment where they’re found.
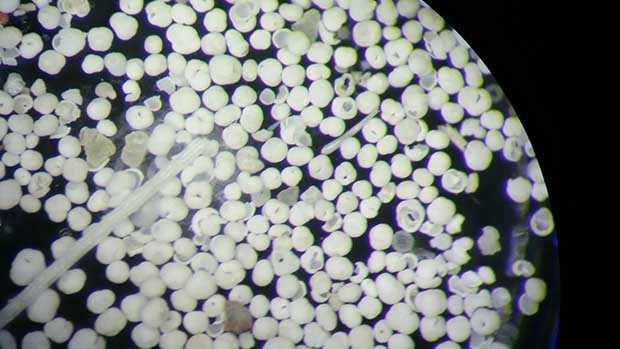
Diatoms
While very common in the ocean, diatoms actually live all over the planet, pretty much anywhere there’s water. They build a skeleton, called a frustule, from silica (SiO2), a combination of silicon (Si) and oxygen (O). Like the calcium carbonate tests of foraminifera, diatoms’ silica frustules can be persevered in ocean sediments for many millions of years.
There are tens of thousands of different species of diatoms, some of which live in very particular environmental conditions. For example, some species live only in areas of the ocean that are covered by sea ice, and others live only in open ocean waters. As with forams, variations in the species of diatoms found in mud layers can tell scientists a lot about past oceanic environmental conditions, and the presence (or absence) of particular species can help date the mud and the environmental record that mud contains.
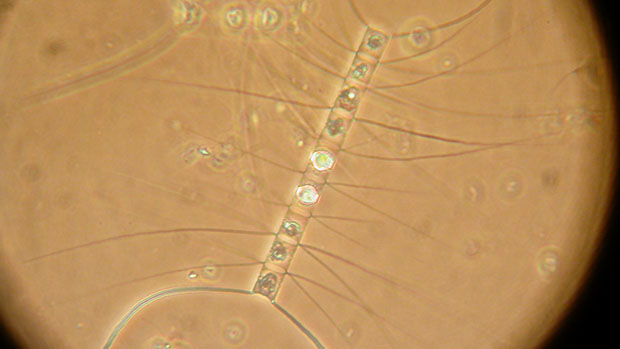
Radiolarians
Radiolarians (nickname: 'rads') also build elaborate skeletons from silica. There are hundreds of species, some of which live in fairly specific conditions within the ocean, so they can be used to track different ‘pockets’ of ocean water as it circulates around the globe. The presence of particular species within mud can tell scientists about possible changes in oceanic currents. They also evolve rapidly which makes them very useful for biostratigraphy.
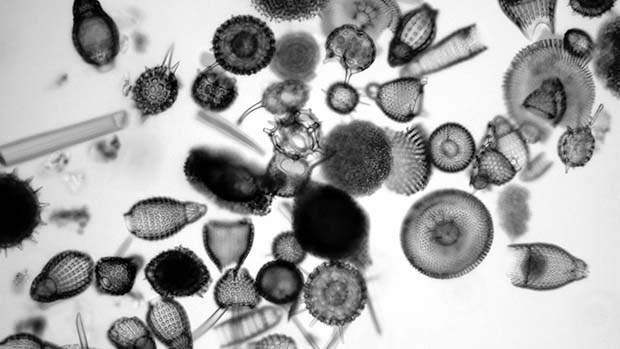
Essentially, the mud—and its microfossils—at the bottom of the ocean is a treasure trove of information about past climates and environmental change.
Getting down and dirty: coring the seafloor
But hang on. These layers of mud are lying on the ocean floor, under hundreds or even thousands of metres of water. We certainly can’t go down in a scuba suit to dig through them where they are, so how do scientists get them to the surface to study them?
It’s done using a process called coring. This involves sending a big metal tube off the back of a ship down to punch through the layers of mud on the ocean floor, capture them and bring them to the surface. There are a few different ways to do this, but the end result is the same—a tube full of sediments that preserves the original order of the layers’ deposition.
This may sound straightforward, but it’s actually a pretty intense process that is a strange mixture of brute force and intricate precision, not to mention an emotional rollercoaster that takes the scientists on a ride through stress and anticipation to excitement to euphoria.
In the first place, ship time is in high demand, and it’s a big deal when you finally get your turn to head out to sea. Depending on where you’re going, you can be out at sea for weeks, even up to months. And because time is so precious, you’ll generally be working 12-hour shifts, tag-teaming with people so that the work can get done around the clock—it’s not just sediment coring, a voyage at sea involves more a whole range of different experiments and sampling so as to make the best use of ship time.
And there’s a few things that can go wrong … manipulating machinery that weighs several tonnes (it has to be heavy to punch metres deep into layers of clay) isn’t easy at the best of times, let alone out in the middle of the ocean. Also, figuring out how much cable to release so as to punch through the ocean floor while not letting out so much that it ends up in a tangled mess at the bottom of the ocean can be tricky. Add to this a ship heaving up and down in the ocean swell and it becomes even trickier! And while there are various tools available that provide information about the ocean floor as you sail over it, you can never be 100 per cent sure exactly what sort of sediments are lying deep beneath—a core barrel punching through sand (rather than sticky clay) doesn’t really get much purchase on the sediments and it can come up empty. And if your core barrel happens to smack into a rock on its way through the sediments, well, that's really not helpful.
All of these factors can make the wait for the core barrel (and this wait can be hours, depending on the depth of the water) to be winched back on board the ship extremely nerve-wracking. But it’s all worth it, when the core barrel finally emerges from the water, with a nice coating of mud on the very top, indicating that it punched through and buried itself all the way into the sediments. Even better is the moment of removing the cover of a core barrel to reveal a beautiful core chock-full of green and grey clays and other sediments, maybe with a layer of sand or a sprinkling of ice rafted debris!
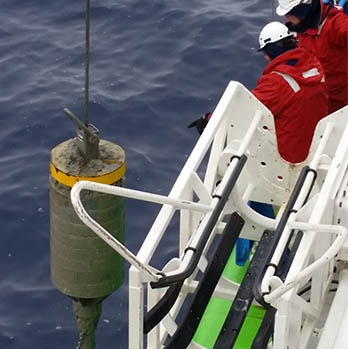
Some methods only retrieve comparatively short cores—around a few metres long—these are ones opened up straight away on the ship for a preliminary examination and small samples of the mud are packaged up to take back for laboratory analysis. Other commonly used coring methods can pull up to 60–70 metres of sediments up from the ocean floor.
The longer cores are often packed up on the ship and not looked at until back in the lab. Imagine the anticipation of having tens of metres of mud that potentially go back millions of years in Earth’s history but having to wait weeks or even months to be able to look at it!
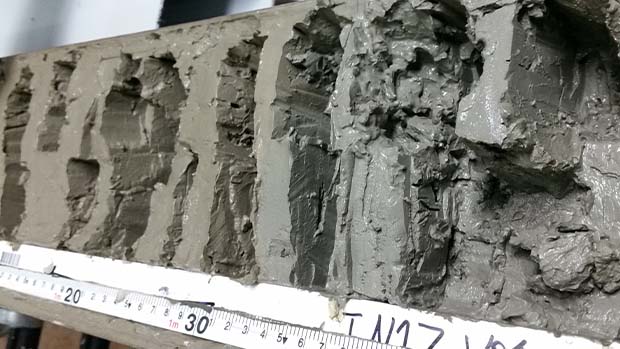
Even longer records can be obtained by using drilling equipment on the specialised ships of the International Ocean Discovery Program to drill down deeper into sediments. These drilling techniques can be used in waters deeper than 5000 meters and obtain a series of shorter cores that when strung together, can capture more than a kilometre of sediment and rock.
The longest sediment record that has been retrieved from the seafloor is nearly 2.5 kilometres long! Even though this core is the longest, the deepest sediments in it are only a little over 20 million years old—quite young, geologically speaking. The oldest sediment record recovered is only about 500 metres long, but dates back around 170 million years.
Telling the story of the mud
With cores that can be opened up and examined on the ship, one of the first things scientists do is look closely at the colour of the sediments. This can range from greenish greys to yellowish browns. Then they rub a small piece of sediment between their fingers—a rough and ready way to feel the texture and tell if you’ve got sand, silt or clay, or sediment rich in microfossils like diatoms or foraminifera.
Mud that is chock-full of diatoms has a spongy, fluffy appearance, and feels smooth. Or, if the mud feels gritty and grainy, this indicates that there’s sand or other material incorporated into the mud as well. It can also be lumpy and chunky like cottage cheese, which indicates that it contains small lumps of hard clay that have been squished and transported beneath a glacier.
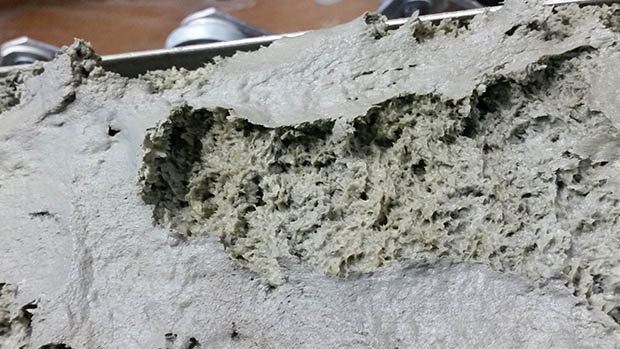
The other features scientists look for are any structures within the sediment: if the layers are all flat and parallel, or if they’re sloping on an angle, or look like they’ve been disturbed and mixed up. They also look to see if there are any fossils or larger pieces of rock (the ice-rafted debris) within the layers.
For ‘young’ sediments—around 50,000 years or less—carbon dating methods can be used to figure out their approximate age. This can be done by analysing the carbon within pieces of shell material or foraminifera, or by collecting mud rich in other carbon-containing organic matter. For older sediments, it’s mainly biostratigraphy methods that are used to determine their age.
Back in the lab, the longer cores are carefully and painstakingly examined, centimetre by centimetre, again noting the changes in the sediment types and structures. Extra geochemical analyses are carried out to provide as much information as possible about the core’s sediments, and the processes that created them. The end result will be a record of climatic change that potentially dates back millions of years.
The amount of information that can be teased out from a sediment core is pretty extraordinary. So next time you squelch your toes through some squishy mud, spare a thought for where that mud might end up in a few hundred thousand years' time and what story it might have to tell!