Printing the future: 3D bioprinters and their uses
Expert reviewers
Professor Gordon Wallace FAA FTSE
Director of the Intelligent Polymer Research Institute
University of Wollongong
Essentials
- Bioprinting is an extension of traditional 3D printing.
- Bioprinting can produce living tissue, bone, blood vessels and, potentially, whole organs for use in medical procedures, training and testing.
- The cellular complexity of the living body has resulted in 3D bioprinting developing more slowly than mainstream 3D printing.
- Bioprinting technology could provide the opportunity to generate patient-specific tissue for the development of accurate, targeted and completely personalised treatments.
- There is still a long way to go before we can create fully functioning and viable organs for human transplant.
The artificial creation of human skin, tissue and internal organs may sound like a futuristic dream but, incredibly, much of it is happening right now, albeit still in the early stages. In research facilities and hospitals around the globe, advancements in 3D printing and, more particularly, bioprinting are providing new options for treatment and scientific study. Indeed, bioprinting has the potential to be the next big thing in health care and personalised medicine.
Biofabrication can be defined as the production of complex living and non-living biological products from raw materials such as living cells, molecules, extracellular matrices, and biomaterials.Mironov et al., 2015.
But what exactly is bioprinting? How does it work? What can it do and what can it really be used for? Let’s take a look …
First things first … understanding 3D printers
Traditional printers—like the type you have in your home or office—work in two dimensions. That is, they are able to print text or images on a flat surface (usually paper), using the x (horizontal) and y (vertical) dimensions. 3D printers add another dimension—depth (z). They can move up and down, left and right, and backwards and forwards and, instead of delivering ink on paper, they distribute different materials—ranging from polymers (including plastics), metal, ceramics, even chocolate—to ‘print’ an item layer by layer in a process that is known as ‘ additive manufacturing GLOSSARY additive manufacturingA process by which digital 3D design data is used to build up a component in layers by depositing material. The term ‘3D printing’ is increasingly used as a synonym for additive manuafcturing. ’.
To create a 3D object, you first need a blueprint—that is, a digital file created using modelling software. Once created, the computer-generated model is sent to the printer. Your chosen material (such as plastic) is loaded into the device, ready to be heated to allow it to easily flow from the printer nozzle. As it reads the blueprint, the printer head moves up and down, side to side and forward and back, depositing successive layers of the chosen material to build up your final product. As each layer is printed it transforms into a solid form, either by cooling, chemical reaction (often induced by light), or by the mixing of two different solutions delivered by the printer head. New layers adhere to the previous one to create a stable, cohesive item. Almost any shape can be created in this way, including moving parts and complex layers.
A wide range of items are already being created using 3D printers, including jewellery, clothing, toys, prototypes, camera cases, and high-end manufactured items.
Bioprinting
Bioprinters work in almost the exact same way as 3D printers, with one key difference. Instead of delivering materials such as plastic, ceramic, metal or food, they deposit layers of biomaterial, that may include living cells, to build complex structures like blood vessels or skin tissue.
Hang on …living cells? Where do they get those? Well, every tissue in the body is naturally made up of different cell types. So the required cells (kidney cells, skin cells and so on) are taken from a patient and then cultivated until there are enough to create the ‘bio-ink’, which is loaded into the printer. This is not always possible, so, for some tissues, adult stem cells—which can develop to form the cells required in different tissues—can be used.
Following detailed computer designs and models, often based on scans taken directly from a patient, precision printer heads deposit cells exactly where they are needed and, over the course of several hours, an organic object is built up using a large number of very thin layers.
Of course, you generally need more than just cells, so most bioprinters also deliver some sort of organic or synthetic ‘glue’—a dissolvable gel, collagen scaffold or other type of support that the cells can attach to and grow on. This helps them to mould and stabilise into the correct form. Amazingly, some cells can assume the correct positioning by themselves with no scaffolding.
How do they know where to go? They use their inherent properties to seek out similar cells to join with. They innately know where they are needed, like the way cells in an embryo develop in the womb, or tissue in an adult moves to repair damage. Researchers are then able to control the shape in which they do that, with the printer building the final structure.
There are many different printers on the market and undergoing testing, and therefore methods for delivering these cells and biomaterials also differ. Some of the more commonly used methods at present include extrusion GLOSSARY extrusionTechnique where continuous filaments of a material are forced through a nozzle in a controlled manner to construct a 3D structure , laser GLOSSARY laserIn laser-guided direct writing method, photons from a laser beam trap and guide cells by exploiting the differences in refractive indexes of cells and cell media. , microvalves GLOSSARY microvalvesThe bioink droplet is generated by the opening and closing of a microvalve under constant pneumatic pressure. , inkjet GLOSSARY inkjetDrop-based bioprinting (inkjet) creates cellular constructs using individual droplets of a designated material, which may also be combined with a cell line. and tissue fragment GLOSSARY tissue fragmentThe combination of bioprinting techniques with biological self-assembly. This approach exploits the intrinsic capacity of closely spaced tissue fragments to fuse together, otherwise known as tissue fluidity. printing.
Each method has its own advantages and drawbacks, and each presents unique challenges to be overcome. Researchers predict it will be the combination of several of these techniques that will provide the most significant advancements in bioprinting. A more detailed explanation of these methods is available from the University of Wollongong.
So, essentially, scientists and medical researchers are using scanners and printers traditionally reserved for auto design, model building and product prototyping to instead create living human tissues.
Universities, researchers and private companies around the world are all involved in advancing bioprinting technologies. Let’s take a look at some of the things they’re working on.
Bone
Every year, more than 2.2 million people worldwide require bone-graft procedures to repair bone defects.
Current bone grafts often use a synthetic cement-based material combined with a patient’s own bone. Limitations with these materials have resulted in structures that do not have appropriate mechanical integrity or allow for the creation of new tissue. Replicating the naturally occurring bone–cartilage interface has also been problematic.
However, a team from Swansea University has developed a bioprinting process that can create an artificial bone matrix in the exact shape of the bone required, using a biocompatible material that is both durable and regenerative. These newly printed ‘bones’ are transplanted into the body where, over a period of several months, they fuse with and are eventually replaced by a patient’s natural bones with few, if any, complications.
To print a small bone with
trabecular
GLOSSARY
trabecularCancellous bone, also known as spongy or trabecular bone, is one of the two types of bone tissue found in the human body. Cancellous bone is found at the ends of long bones, as well as in the pelvic bones, ribs, skull and the vertebrae in the spinal column.
features currently takes around two hours. With these short time frames, surgeons could soon be printing them in the operating theatre as they work.
Other researchers at the University of Nottingham in England are working on similar bone replacements. They bioprint an exact copy of the part of the bone they are trying to replace—this becomes the scaffold. This is then coated with adult human stem cells—capable of developing into almost any different tissue type. This is combined with bio-ink from the printer—a combination of polylactic acid (which provides the mechanical strength of bone) and alginate—a gel-like substance that works as a cushioning material for the cells. The final product is then implanted into the body, where, within about three months, the scaffold will disappear and be replaced by new bone.
Researchers hope that, in the future, bioprinted bones could be created with enough reliability to underpin intricate spinal reconstruction, and that the bone material could be further improved to enhance its compatibility with cartilage cells.
Skin
If a person is badly burned, healthy skin can be taken from another part of the body and used to cover the affected area. Sometimes, however, there is not enough undamaged skin to harvest.
Researchers working at the Wake Forest School of Medicine have successfully designed, built and tested a printer that can print skin cells directly onto a burn wound. A scanner first determines the size and depth of the wound, and this information is passed to the printer, which, after the relevant cells have been cultivated, applies the correct cell types at the correct depth to cover the wound. Unlike traditional skin grafts, you only require a patch of skin one-tenth the size of the burn to be able to grow enough skin cells for skin printing. While this technology is still in the experimental stage, researchers hope it will be widely available within the next five years.
As mentioned, 3D printers print in layers, and because skin is a multilayered organ with different cell types, it’s well suited to this type of technology. One American company has already produced multilayered skin consisting of the dermis and epidermis layers. There are still many challenges ahead though, particularly how to stop the heat generated by the printer from damaging the cells or their viability. And of course, as with most parts of the human body, skin is more complex than it first appears—there are nerves, blood vessels, and a myriad of other aspects that need to be accommodated.
Blood vessels
Considering there are tens of thousands of kilometres of veins, arteries and capillaries in your body, it’s good news that researchers have been working on ways to replace them should they ever fail. Creating viable blood vessels is also essential to get all those other potential bioprinted body parts to work properly.
Research engineer Monica Moya from Lawrence Livermore National Laboratory is using bioprinting to create ‘living’ blood vessels. The materials and environment created by her bioprinters are engineered in such a way as to enable small blood vessels—human capillaries—to develop on their own. This development takes a while so, to assist, tubes of cells and other biomaterials are printed out to help deliver vital nutrients to the surrounding printed environment. Over a period of time, the self-assembled capillaries connect with the bioprinted tubes, thereby beginning to deliver nutrients to the cells on their own—mimicking the way these structures work in the human body.
If you take this approach of co-engineering with nature you allow biology to help create the finer resolution of the printed tissue … We’re leveraging the body’s ability for self-directed growth, and you end up with something that is more true to physiology. We can put the cells in an environment where they know, ‘I need to build blood vessels.’ With this technology we guide and orchestrate the biology.Monica Moya, Research engineer
Having successfully created an ‘unorganised’ network of blood vessels, she and her team are now focusing on creating a tiered hierarchy such as that which exists in the human body.
Another method of creating blood vessels has been developed by researchers at the Brigham and Women’s Hospital. Using an agarose fibre template covered with hydrogel, the team was able to construct microchannel networks which exhibited various architectural features.
Internal organs
There are currently around 1,600 people on the organ transplant waiting list in Australia. Many researchers hope that, within 20 years, these lists will be a thing of the past. They imagine a world in which an organ can be printed and transplanted in just a few hours, without rejection from the body. These printed organs will be created from the very cells of the body they will re-enter, matching the exact size, specifications and requirements of each individual patient.
At present, the bioprinting of fully functional complex internal organs (such as hearts, kidneys and livers) is still at least 10 years away, possibly more, but advancements are occurring at a rapid rate.
Less complex organs—such as bladders—are already possible. At the Wake Forest University in the United States, researchers have successfully taken cells from a patient’s original, poorly functioning bladder, cultivated them and added additional nutrients. A 3D mould of the patient’s bladder was then printed and the cultivated cells soaked through it. The mould was placed in an incubator—a bit like baking a cake—and, when it came out, was able to be transplanted into the patient’s body. The mould will eventually break down, leaving only the organic material behind.
The same team have also successfully produced viable urethras. Once again, a combination of a patient’s own cells and additional nutrients is seeded with scaffolding and then sewn into the correct shape of a urethra. These engineered parts can then transplanted back into the patient’s body.
While bioengineers have successfully printed a tiny (1 millimetre thick x 4 millimetres wide) 3D functioning liver, it survived for only five days. It’s a start, but there’s a long way to go.
Kidneys are also incredibly difficult as they contain complex cellular processes for filtration—not something that can be easily replicated. Researchers in Australia have used human stem cells to grow a kidney organoid that contains all the required cell types for a kidney. Such cells may provide a valuable starting source for bioprinting a more complex kidney structure.
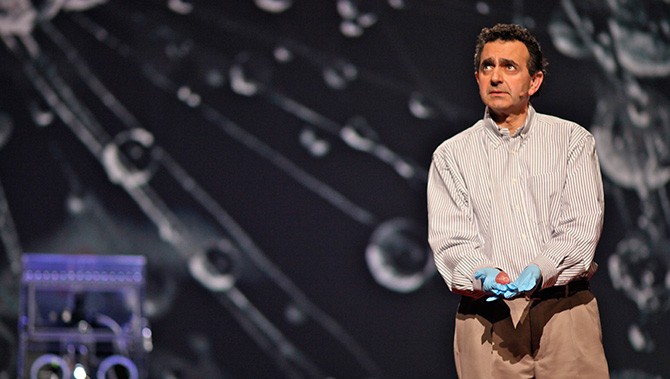
Amazingly, hearts may be one of the easier organs to make, as they’re essentially a pump with tubes. Of course, it’s not quite as simple as that, but many researchers believe that we’ll have transplantable biohearts before kidneys or livers. Researchers in the United States have created ‘organoids’—3D printed, beating cardiac cells, which are shaped using a specialised 3D printer and are, essentially, fully functional.
While it’s easy to print a full replica of an organ, it’s more difficult to make it ‘real’, with blood vessels, connecting tissues, nerves, filtration systems, strength, durability and all the aspects that go in to making our ‘natural’ organs so complex. These challenges aren’t putting off the many researchers who are working to find solutions to these intricate problems.
Human cartilage
The 3D printing of human cartilage should, researchers hope, lead to implantable replacements for trauma victims who need reconstructive surgery. Ear sliced off? Nose smashed? No worries—scientists in Zurich have developed a process that could enable hospitals to print a full-sized human nose implant in under 20 minutes. They believe that any cartilage implant could be produced with their new process. In an article, researcher Matti Kesti described the technology:
‘A serious car accident results in a passenger’s nose being shattered. It is possible to reconstruct this as a 3D model on the computer. At the same time, a biopsy is performed on the patient and cartilage cells removed from his or her own body, for example from the knee, finger, ear or splinters of the shattered nose. The cells are spawned in the laboratory and mixed with a biopolymer. From this toothpaste-like suspension, a nose cartilage transplant is created using the bioprinter, which is implanted in the patient during surgery. In this process, the biopolymer is used merely as a form of shaping mould; it is subsequently broken down by the body’s own cartilage cells. After a couple of months, it is impossible to distinguish between the transplant and the body’s own nose cartilage.’
As the implant was grown from the body’s own cells, the risk of rejection is far lower than for an implant made of, say, silicone. An added benefit is that the cellular implant grows together with the patient, because it is controlled by the patient’s internal growth engine, as is the case for other body parts. This is particularly important for younger implant-recipients.
A bonus use: drug testing and medical research
One of the key potential areas for using bioprinted living materials is in the arena of medical testing, drug research and safety. Many of these bioprinted tissues share common features with native tissues—such as the presence of multiple cells types, cellular density and key architectural features. In this way, researchers are able to study the effects of different diseases, disease progression and possible treatments in a native microenvironment.
One of the most exciting recent advances is the idea of a ‘benchtop brain’, which has been developed by researchers at the ARC Centre of Excellence for Electromaterials Science (ACES). They have developed a 3D printed, six-layered structure that incorporates neural cells that mimic the structure of brain tissue.
This has huge potential benefits for researchers, pharmaceutical companies and private companies. It will enable them to test new products and drugs on tissue that accurately reflects human brain tissue, as opposed to animal specimens which can produce a very different reaction. The benchtop brain could also be used to further research into brain disorders such as schizophrenia or Alzheimer’s disease.
We are still a long way from printing a brain but the ability to arrange cells so as they form neuronal networks is a significant step forwardACES Director and research author Professor Gordon Wallace
By allowing researchers to work with ‘real-time’ human tissue, it could greatly speed up testing processes, and produce more realistic, accurate results. It could also help reduce the need to use lab animals for medical tests, as well as potentially dangerous trials on humans.
Mobile 3D printing
A spin-off from the traditional bioprinter is the BioPen, developed by Australian researchers and led by Professor Gordon Wallace at the University of Wollongong. The pen functions in a similar way to a bioprinter, but is far more mobile. It combines cellular material with protective gels, which are delivered via the pen head as the surgeon ‘draws’ with the ink directly on the damaged segment of bone or cartilage to repair any damage. As each layer is ‘drawn’ by the surgeon, it is exposed to UV light from a source attached to the pen. This hardens the gel so that additional layers can be added, eventually creating a three-dimensional framework.
The pen will give surgeons a greater degree of accuracy and control over where they place biomaterial, reduce time in surgery and accelerate the regeneration of bone and cartilage. Once the biomaterial is delivered to the exact site determined by the surgeon, the protective gels gradually degrade away, replaced by the new tissue which begins to repair the area.
Professor Peter Choong, BioPen co-developer and director of orthopaedics at St Vincent’s Hospital Melbourne, has been using the pen in clinical trials since 2013. He noted, ‘Professor Wallace's research team brings together the science of stem cells and polymer chemistry to help surgeons design and personalise solutions for reconstructing bone and joint defects in real time’.
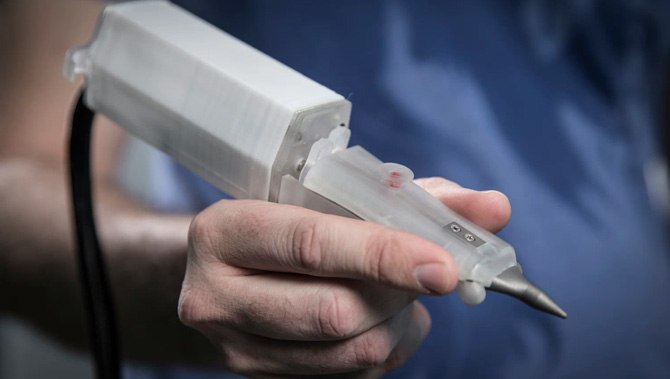
Why is it taking so long?
The human body and its various components are a lot more complicated than a plastic toy or a ceramic piece of jewellery. A human organ has a complex web of cells, tissues, nerves and structures that need to be correctly positioned for the organ to function properly. From arranging the thousands of tiny capillaries in a liver, to actually getting a printed heart to ‘beat’ and contract—it’s a long, difficult process. While some parts of the human body are more complex than others—an ear or nose is easier to make than a bladder or a urethra, while the larger organs like kidneys, heart and liver are at the top of the difficulty rating—each piece has its own specialised requirements and issues that need addressing. Added to this is the selection and availability of the right materials, cell types, and creating bio-inks that meet the dual demands of both printability and cell functionality. There is also the need to take in to account different growth rates and differentiation factors, as well as developing the right software and hardware to make it all come together.
Even after researchers believe they have it all ‘right’, bioprinted technologies—like all new medical treatments—are still required to pass safety tests and proper regulatory processes before they can be made available to the public.
Working through all these complexities requires the integration and input of technologies from fields including engineering, biomaterials science, cell biology, physics and medicine. So, you’ll just have to be a little more patient!
Conclusion
Given its interdisciplinary nature, 3D bioprinting is accelerating at an ever-increasing rate. It’s exciting times, however, we need to be careful to temper our expectations of this technology with the realities. The human body is incredibly complex, and trying to replicate the many things that it does is difficult, challenging work. Those working in the field are making advances every day, in both the technology and in their understanding of how it can be used and improved. While we’re not quite there yet, there is no doubt that the future of medicine will be very different with bioprinting involved.