Feeling the heat: geothermal energy
Expert reviewers
Professor Suzanne O'Reilly AM FAA
Director, ARC Centre of Excellence for Core to Crust Fluid Systems and Earth and Planetary Sciences
Macquarie University
Essentials
- Geothermal energy is heat from within Earth
- Geothermal energy is a clean, renewable resource which can help reduce the effects of climate change
- Geothermal energy can be used directly in industrial or domestic applications, or indirectly to produce electricity
- There is enough geothermal energy in Earth to provide for all our power needs for billions of years
- While well developed in other countries the Australian geothermal industry is still in its infancy
Our planet is feeling the heat—but it’s not all from climate change and the increasing concentration of greenhouse gases in the atmosphere. Some of the heat is coming from within Earth itself; and rather than causing global warming it has the potential to wean us off fossil fuels for good.
What is geothermal energy?
Geothermal energy is heat from within Earth. It is a clean, renewable resource and it lies in abundance beneath our feet. It can be used directly (for industry, agriculture, heating and cooling) or indirectly to generate electricity. If the energy stored in hot rocks inside Earth could be tapped and used instead of fossil fuels, it could help to reduce the threat of climate change.
The deeper you go into the planet, the hotter it gets. Part of the heat is left over from the creation of Earth, which started off as a hot cloud of gas and dust and has been cooling over time. Earth's outer layers have cooled most quickly, forming the crust; heat from the core continues to radiate outwards, like any cooling object. Heat flows from hot to cold—Earth's inner core is hotter than 5,000°C, while the surface is generally less than 30°C and outer space is close to absolute zero. The rate of change of temperature with increasing depth in within Earth is called the geothermal gradient GLOSSARY geothermal gradientthe rate of increasing temperature with respect to increasing depth in the Earth's interior. Away from tectonic plate boundaries, it is about 25 °C per km of depth in most of the world. .
There is another source of geothermal energy: radioactive decay. Naturally occurring radioactive elements break down into more stable elements by emitting small amounts of radiation. Low concentrations of uranium, thorium and potassium in certain types of rocks decay over very long periods of time. The radiation they emit as they decay heats the rocks in which they are contained, creating a localised increase in temperature (a thermal anomaly). This ongoing generation of heat by radioactive decay and the continuous flow of heat towards Earth’s surface are reasons geothermal energy is considered a renewable resource.
There seems to be enough geothermal energy to keep us all ‘rocking’. Earth’s total heat content has been calculated at 12.6 x 1024 megajoules, which would meet the world’s current energy needs for several billion years. Unfortunately, most of this is inaccessible; but tapping just a fraction of it would make a substantial contribution in terms of reducing greenhouse gas emissions. It has been estimated that in Australia, using just 1 per cent of the geothermal energy shallower than 5 kilometres and hotter than 150°C could supply all our energy requirements for 26,000 years (and then some, as this figure does not take into account geothermal energy’s renewability). Geothermal exponents talk about an ‘almost limitless’ supply of energy. The challenge is tapping into it in a cost-effective way.
Where is geothermal energy found?
Geothermal energy is, theoretically, everywhere, although high temperatures are closer to the surface and more obvious in some places than others. Some visible signs of geothermal energy include hot springs, geysers, volcanoes and fumaroles, but the majority is hidden deep underground. To find geothermal resources, geologists use a variety of methods and techniques. These include studying aerial photography and geological maps, testing the chemistry of local water sources and analysing the level of certain elements in the soil, checking variations in gravity and magnetic fields, and measuring the geothermal gradient. Despite even the most rigorous research, the only way to truly test a geothermal resource is to drill wells and measure the temperature and other geological conditions at the depth of the resource.
Types of geothermal energy
Geothermal resources vary in character and in the ways in which they can be used. As previously mentioned, geothermal energy can be used for either direct-use applications or to produce electricity. Generally lower temperature (less than 150°C) resources can be best used in direct-use applications while high temperature resources are more frequently harnessed to produce electricity.
The temperature of the geothermal resource is just one characteristic that can be used to classify the type of resource. The range of geothermal resources can be thought of as a continuum with variations in the temperature and the ability to naturally flow water through the rock (permeability). The variations in the type of resource lead to variations in the way in which they are used. Broadly they can be classified into four types of resources, and only some of these are found in Australia.
Ground Source Heat Pumps
Ground Source Heat Pumps are a type of direct-use application that uses the constant temperatures found at shallow depths below ground for the heating and cooling of buildings. Pipes are buried in either trenches or deeper holes (usually less than 100 metres deep) and fluid is circulated through these pipes. During summer the ground is cooler than the air temperature and vice versa in the winter months.
Ground Source Heat Pumps can be used almost anywhere and can provide a very efficient method of heating and cooling buildings. In some countries, such as Norway, this system has become a standard installation in new domestic, business and industrial constructions for year-round temperature control.
Hot Sedimentary Aquifer
In certain areas naturally occurring groundwater is available which, due to the geothermal gradient, is naturally heated. This water can be extracted from the aquifer GLOSSARY aquifera body of permeable rock which can contain or transmit groundwater. and used for a variety of applications depending on the temperature and available volume. For lower temperature aquifers, the most efficient application is to use the heat directly rather than converting it to electricity. For example, many places around Australia use warm groundwater sources to heat public swimming pools. In many of these cases once the water has been used in the heating application it is re-injected back in to the ground to ensure the sustainability of the resource.
In other places around the world these types of resources are used for a variety of industrial applications such as heating in aquaculture and greenhouses, water-desalination or drying industrial products. There are also various industrial applications at higher temperatures.
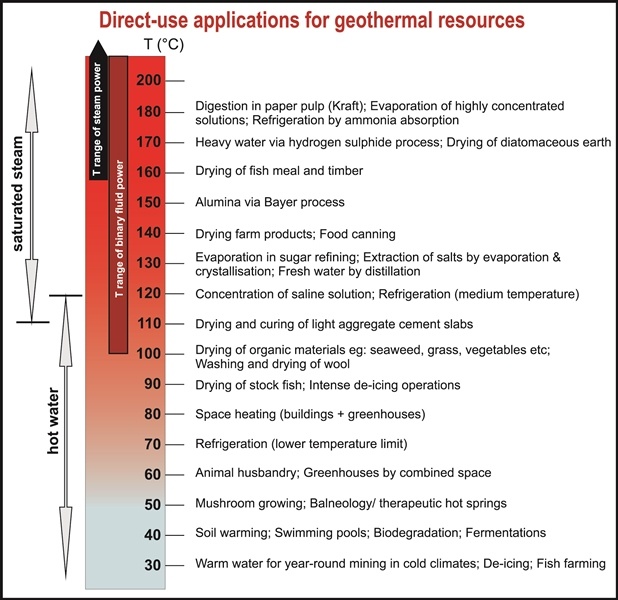
The Lindal diagram shows how geothermal energy resources of different temperatures can be used for a range of applications. Credit: Geoscience Australia
Groundwater at temperatures greater than around 100°C can be used to produce electricity, although the efficiency of electricity generation (and therefore, the economic viability) will increase with higher temperatures. The hot water in these systems can be extracted from the aquifer either by pumping or, in some cases, it flows to the surface under artesian GLOSSARY artesianrelating to or denoting a well bored perpendicularly into water-bearing strata lying at an angle, so that natural pressure produces a constant supply of water with little or no pumping. conditions. The hot water is used to heat a secondary fluid in the power plant which is then used to drive the turbine to produce electricity. This type of power generation is known as a Binary Plant. Australia’s only operating geothermal power station at Birdsville in Queensland uses this type of resource.
Hydrothermal Systems
The majority of geothermal power production around the world uses hydrothermal resources. These resources are generally very high temperature (greater than 250°C), relatively shallow (less than 1,000 metres), and have the ability to naturally flow fluid through the rock at high volumes (high permeability). They are generally found in volcanic or tectonically active regions of the world such as Iceland, western USA, Japan, New Zealand, African Rift Valley, the Philippines and Indonesia. They often have surface expressions such as hot springs or geysers. The water in these systems is heated by either volcanic activity or by the fact that hotter regions are closer to the surface because movement of tectonic plates results in the crust is naturally thinner in these areas.
Due to their surface expressions and high temperatures, these were the first systems to be used to generate power.
The heat from these systems is often harnessed, keeping the fluid under pressure until it is delivered directly to the power plant and then allowing it to ‘flash’ to stream and drive the turbine. These power generation systems are known as ‘Flash Plants’.
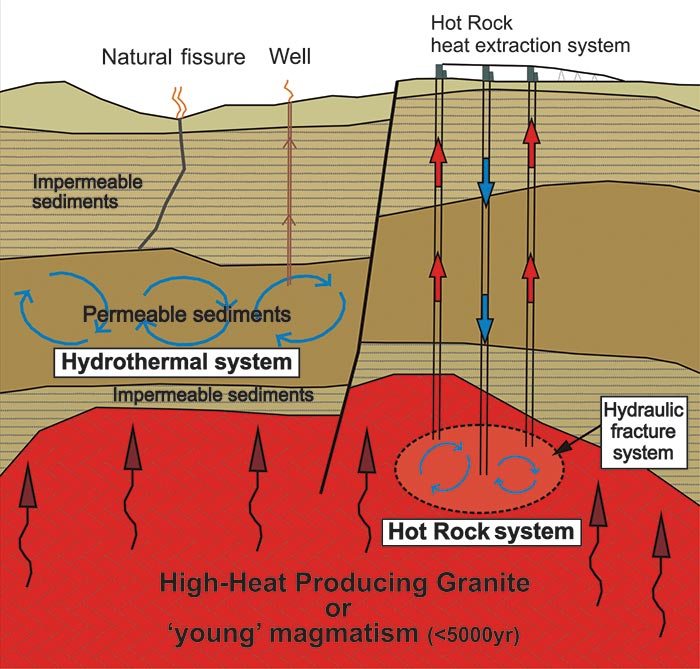
Enhanced Geothermal Systems (EGS)
In many cases, an area within Earth may have elevated temperatures but, due to a number of conditions may have very limited ability to flow fluid through it (low permeability) in order to be able to extract the heat. In these cases the system can be ‘enhanced’ to improve the permeability and in some cases water can be introduced to ‘dry’ systems.
Improved hydraulic fracturing technology has recently made this form of geothermal energy more accessible. The process fractures rocks at depth, allowing water to be injected down a well into the rock formation. A second well is drilled to extract the heated fluid so that it can be used to generate electricity. Binary power generation systems are generally used in EGS to provide greater control over the internal conditions of the power plant and to avoid loss of fluid for the reservoir GLOSSARY reservoira large natural or artificial lake used as a source of water supply .
These systems most often rely on radiogenic GLOSSARY radiogenicproduced by radioactivity heat production being trapped by layers of other rock with low thermal conductivity, like a warm blanket. As a result of these requirements they are most often quite deep (4,000–5,000 metres). This depth can present extra challenges in developing the system and also increase costs dramatically.
Let’s take a look at the two most common systems used in Australia:
Hot Sedimentary Aquifer (HSA) systems are found in sedimentary basins that have retained natural permeability. The water is contained within, and moves through, the rock in the pore GLOSSARY porea minute opening in a surface, especially the skin or integument of an organism, through which gases, liquids, or microscopic particles may pass. spaces between the grains that form the rock, much like water in a sponge. These systems in Australia are most often heated by radiogenic rocks, such as certain types of granite, at depths below the aquifer. Heat is extracted by bringing the heated water to the surface via holes drilled into the aquifer. In the majority of cases the water needs to be reinjected to ensure the sustainability of the system. The only operating geothermal power station in Australia, at Birdsville in Queensland, is an example of a hot sedimentary aquifer system. In Birdsville, after the water has been used to generate power, it becomes part of the town’s water supply.
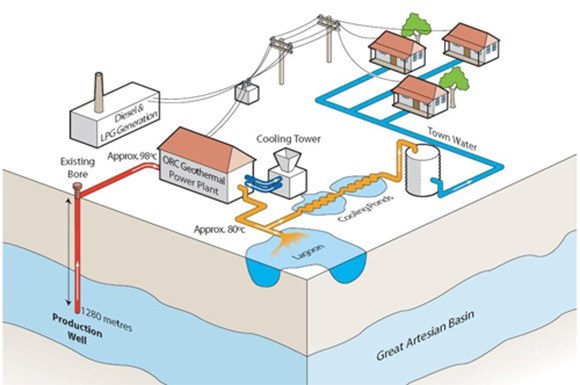
Enhanced/Engineered Geothermal Systems
The term Enhanced (or Engineered) Geothermal Systems (EGS) covers a range of geological systems that can be developed to use geothermal energy. In these systems the one consistent characteristic is the presence of abnormally high temperatures at accessible depths, however other elements of the geothermal system may vary. The system will require enhancement or engineering in order to extract the heat to use it.
To do this a borehole is drilled to the depth of the reservoir that is to be developed. Existing fractures in the rock are then expanded by the injection of high pressure fluids. This moves the rock causing a slight displacement, creating increased permeability when the applied pressure is removed. A second borehole is then drilled into the expanded fractures to allow the circulation of geothermal fluids.
Sometimes called Hot Rock (or less accurately, Hot Dry Rock) these systems are the second type of geothermal resource in Australia and hold promise as a major contributor to Australia’s future energy supplies. But Australia’s geothermal resources are only now starting to be understood. Data on rock temperatures down to a depth of about five kilometres are available from nearly 6,000 bore holes drilled during exploration for petroleum and minerals. These bore holes are distributed unevenly across the continent and many are shallower than five kilometres, so the geothermal resource is better understood in some areas than others.
Heat Flow determinations are a robust exploration dataset but are much less common. A Heat Flow determination requires measurement of both the thermal gradient, taken in boreholes drilled in the field and laboratory measurements of thermal conductivity of the rocks from the same borehole. Heat Flow determinations are a measure of the amount of heat (energy) moving through the crust in a given time.
Despite the need for more data, it is clear that Australia has massive geothermal resources. This is partly because of the presence of large quantities of heat-producing granites in the upper crust enriched by higher than normal concentrations of radioactive elements. The Cooper and Eromanga basins in South Australia and Queensland contain geothermal resources that reach temperatures of up to 250°C at 4.5 kilometres below the surface, putting them among the world’s hottest rocks at this depth (excluding volcanic systems).
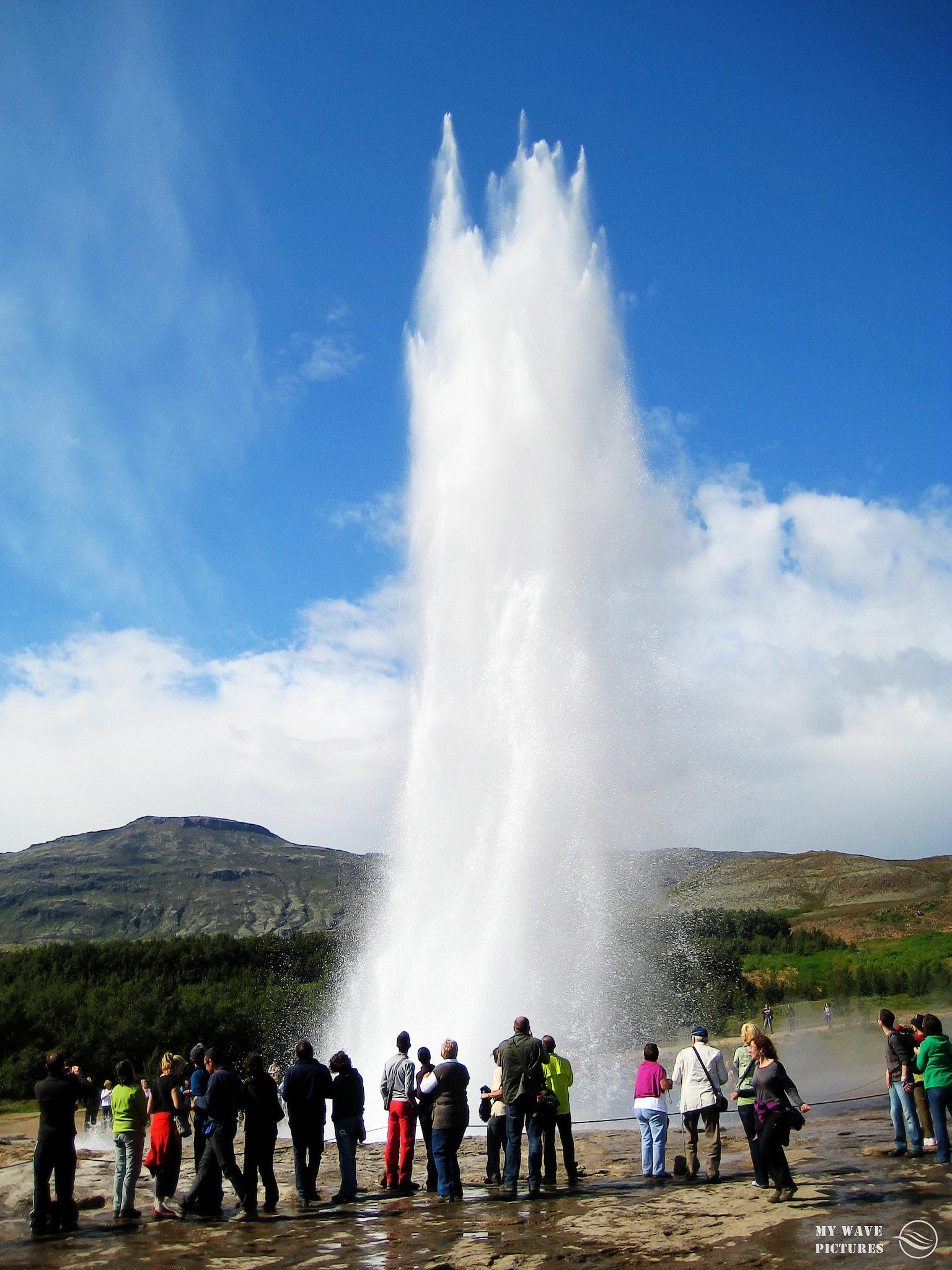
Strokkur (Icelandic for "churn") is a fountain geyser in the geothermal area beside the Hvítá River in Iceland. It erupts about every 4–8 minutes, sometimes up to 40 metres high. Photo credit: My Wave Pictures. Flickr
Using geothermal energy
High temperature geothermal systems can be used for electricity generation, cooler systems can be used for direct-use applications, and shallow ground source heat pumps can be used in a wide variety of geographical locations.
Direct uses
Geothermal resources can be tapped directly for their heat energy. The heat can be used in a wide variety of industrial and domestic applications for example, refrigeration, desalination, heating of fish farms, drying timber and space heating and cooling. In other parts of the world such as Iceland, geothermal energy is used to heat greenhouses that produce year-round fruit and vegetables in an otherwise inhospitable environment. Several locations around Australia are already using geothermal heat to warm swimming pools, spas and buildings.
Geothermal heat pumps, or Ground Source Heat Pumps (GSHP) are already being used to heat buildings around Australia including the Geoscience Australia building in Canberra and Antarctic Tasmania in Hobart. GSHP use the constant temperature of the ground at depth to provide a heating benefit in the winter and a cooling benefit during summer. The depths required for GSHP are much less than other geothermal systems, with installations varying in depth from just a few metres to about 100m. There is increasing interest in large-scale GSHP technology from utility companies to supply heating and cooling for new suburbs and housing estates.
Electricity generation
In Australia, however, perhaps the most exciting potential application of geothermal energy is its indirect use to generate electricity. Most electricity today is generated using heat. In a coal-fired power station, for example, coal is burnt to heat water, which turns to steam, which is used to spin turbines, which generates electricity.
Geothermal heat can be converted into electrical energy in much the same way using dry steam, flash, or binary GLOSSARY binaryrelating to, composed of, or involving two things generation systems.
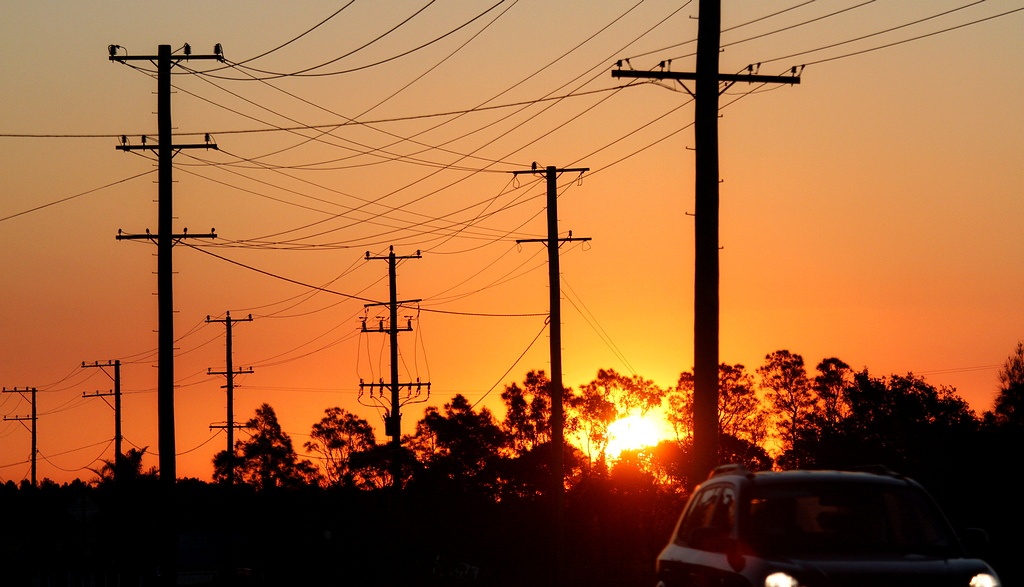
Some countries are already producing electricity from their geothermal resources and have been for decades: the United States, for example, generates around 15 billion kilowatt hour of power per year, equivalent to around 25 million barrels of oil.
Pros and cons of geothermal energy
There are several key benefits to geothermal energy:
Renewable: The heat continuously flowing from Earth’s interior is estimated to be equivalent to 42 million megawatts (MW) of power—ensuring an almost inexhaustible supply of energy for possibly billions of years to come. While the heat of a hot rock reservoir tapped for its energy can be depleted, it will eventually be replaced. If managed carefully, by controlling the flow rate and/or rotating between a number of reservoirs, geothermal resources can be used sustainably.
Baseload: Geothermal energy is available 24 hours a day, 7 days a week, every day of the year. Unlike other renewable energy sources, it does not rely on favourable weather conditions (such as sun or wind), or storage systems, to generate power. A well designed and managed geothermal power plant can also adjust the amount of power generated to match to demand, as it changes depending on the time of day and the seasons.
Domestic: Australia has a range of potential geothermal resources, which if tapped will reduce the need to bring in energy from abroad.
Clean: Modern closed-loop geothermal power plants emit little to no greenhouse gases, life-cycle greenhouse emissions are lower than for solar and natural gas, and geothermal power plants use less water on average than other conventional power generation technologies.
Small footprint: Geothermal power plants are compact and require less land than coal, wind, hydro or solar operations.
Given its benefits, why isn’t geothermal energy already being used commercially everywhere, especially in Australia? There are several reasons for this.
Technical challenges: The techniques for tapping hot rock resources are still being developed. A test project at the Cooper Basin Innamincka site returned encouraging results, but how the properties of hot rock reservoirs might change over time is unknown; such changes might also differ between locations. The combination of the high pressure and temperature of hot rocks in this location caused problems with drilling and well design, but these challenges were overcome.
Start-up costs: Given the lack of existing technologies and the costs of drilling, the start-up costs of hot rock geothermal operations are very high. Geothermal ventures require investors willing to risk their money in technology that is developing. This is particularly challenging in Australia in the current climate of falling power demand, very cheap fossil fuel based power generation, and a lack of subsidies from government.
Infrastructure: As for other renewables including wind and solar, distance from population centres can be a problem. While some of the currently best-understood geothermal resources are a long way from cities there are a number of smaller projects that are pursuing developments closer to electricity markets. Additionally, the Australian resource industry is very familiar with working in remote areas and dealing with the complexities that entails. In some instances it is likely that opportunities for geothermal will arise providing off-grid power to other resource industries such as larger mines or remote communities. Also, having the generation centre some distance from populated areas should reduce concerns over competing land use or other community objections to development.
Contaminants: In some systems, the hot geothermal fluids contain dissolved minerals and gases. Some of these might have commercial value, but there might also be a risk of groundwater contamination and the release of greenhouse gases to the atmosphere. Techniques used in the petroleum extraction industry to minimise potential contamination will be adapted and adopted by geothermal operators. The very small amounts of radioactive substances that occur naturally in rocks are believed to be too low to be of concern. For example, the level of uranium in high heat-producing granite is 100 to 1,000 times lower than in a naturally occurring uranium ore body and about the same as Australian coal.
Careful management of the fluids and gases from a geothermal system is required. The use of closed systems like the one tested at Innamincka, which reinjects any produced fluids into the original reservoir overcomes many of these issues.
Seismic effects: The hydrofracturing process employed in the creation of hot rock reservoirs can induce low level seismic activity—or mini earthquakes. Hydrofracturing experiments in the Cooper Basin, for example, have induced more than 27,000 small earthquakes, although few could be felt at the surface and none were sufficiently strong to cause any damage to nearby infrastructure. Geologists are learning more about the potential risks associated with hydrofracturing and developing strategies to minimise them.
Conclusion
There are good reasons to be excited by the prospects for geothermal energy, both in Australia and world-wide. The international geothermal power market is growing at a sustained rate of 4–5 per cent, with almost 700 geothermal projects under development in 76 countries.
The benefits of geothermal energy—its renewability, low environmental footprint, flexibility for direct-use and power generation and baseload nature will also become of greater value to countries as the effects of climate change become more apparent.
As more money is invested in geothermal energy and its technologies, this should continue to build momentum in the field. Should the technology improve and become more cost-effective, geothermal energy could be set for a rapid expansion. If a sizeable industry develops it will help Australia to keep rocking on.