Concentrating solar thermal
Expert reviewers
Essentials
- Concentrating solar thermal (CST) uses sunlight to produce large amounts of heat.
- The heat is then used to drive chemical reactions or produce electricity, usually by heating water to steam that drives a turbine connected to a generator.
- There are four main types of CST systems—parabolic troughs, linear Fresnel reflectors, solar towers and solar dishes.
- All of the methods use mirrors to reflect sunlight onto a central location, where the concentrated radiation generates large amounts of heat.
- CST can provide large-scale grid level electricity production because heat produced at a plant can be stored without using batteries.
When you think solar power, you most likely think of the solar panels that adorn so many rooftops these days. The type of electricity the solar panels generate is known as solar pv—short for photo (light) voltaic (from volt, which is the unit of measurement of electricity). Solar panels use the energy from the sunlight to directly produce a voltage—the energy from the sun causes a flow of electrons within the materials the panel is made from, which creates an electric current.
There is another type of solar technology that also takes energy from the sun and uses it to produce electricity, but it does it in a very different way. Concentrating solar thermal (CST), also known as concentrated solar power, or solar thermal electric, takes the sun’s radiation and converts it to heat rather than producing an electric current. The heat is then used to produce electricity, or can be stored to use later down the track.
Solar thermal technology has actually been with us for a lot longer than solar pv—think of all the solar hot water tanks and pool heaters that were around long before the proliferation of rooftop solar pv!
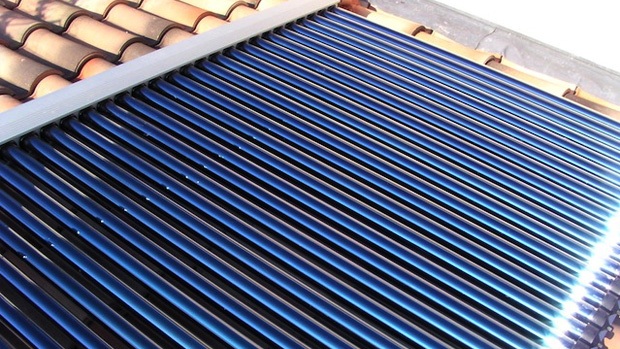
The key to concentrating solar thermal technologies lies in the concentrating part. Sunlight is collected from a very large area and using carefully arranged mirrors, it’s all concentrated upon a single much smaller region called the receiver. The concentrated radiation absorbed by the receiver results in very high temperatures.
The temperature at the receiver can reach up around 3,000°C, however, we’re only able to practically use temperatures of up to around 1,000°C—anything higher would basically cause the receiver to melt! In most cases, the high temperatures heat up a fluid, known as the heat transfer fluid, which is pumped to another region of the plant called the power block. Here the heat is usually used to produce steam from water, and in the case of electricity production, the steam drives a turbine connected to a generator.
Obviously lots of sunlight is needed for a solar thermal plant, and they perform best in arid and semi-arid areas with clear skies. As the system is based on the transfer of heat, it’s also important to minimise thermal losses, so a warm climate is also desirable. The most promising areas are Australia, the Middle East, North Africa, parts of South Africa, US, Chile, Spain, India and the Gobi Desert region of north western China and southern Mongolia.
This map shows the average amount of direct normal irradiance (sunlight that comes from directly overhead) received around the world.
DNI Solar Map © 2016 Solargis.
CST is generally used to generate electricity, but it can also produce heat to drive other useful industrial chemical processes.
An extremely significant aspect CST is that the extra heat that is produced at a plant can be stored. This means CST can provide large-scale grid level electricity production, with the added bonus of storage that other technologies can’t provide without the use of external batteries. It’s easier to store heat than it is to store electricity, so this is a huge commercial advantage. Heated molten salt (see below) can be stored in a giant insulated tank for several hours and used when needed, including at night. It can also be used to adjust the heat (and subsequent electrical) output to meet peak requirements. The molten salt reservoir stores heat energy, similar to the way a battery stores chemical energy—both provide energy storage that’s ready to go when we need it.
Catching the heat
You can build a small solar thermal concentrator that will work in your backyard to cook your sausages and bread, as quick and easy at you please. In terms of wholesale production of electricity that can be distributed through the electricity grid, you need a lot of heat, and to get that heat, you need a lot of mirrors. You also need a lot of space to spread, lay out and arrange the mirrors, and CST plants can cover several square kilometres of ground.
There are a few different engineering techniques used to build large-scale CST systems: parabolic troughs, linear Fresnel reflectors, solar towers and solar dishes.
Parabolic troughs
Long troughs of parabolic (U-shaped) mirrors focus sunlight on a receiver tube placed in the middle of the trough, positioned to catch the focused radiation as it reflects off the mirrors. The sunlight is concentrated by up to 80 times. The mirrored trough modules can be 100 metres long with an aperture (opening/width) of 6 metres or even wider.
The receivers— evacuated glass tubes GLOSSARY evacuated glass tubesFormed by placing a tube inside a glass tube, and ‘evacuating’ the space between them—sucking the air out to create very low air pressure, approaching the state of a vacuum. This space acts as an extremely efficient insulator. Radiation passes through the glass tube, and is absorbed by the inner tube. The lower wave-length infra-red radiation that is then emitted by the surface of the inner tube can not pass through the evacuated space, and so is retained. with black interiors—minimise thermal losses and maximise light absorption. They contain a heat transfer fluid, almost always a high temperature oil, which is pumped through the tube to collect the heat and carry it to an electricity generation plant. Parabolic trough systems generally operate at temperatures around 400°C.
The parabolic shape of the collectors means that all sunlight hitting the surface of the mirrors is reflected to the one focal point in the middle of the parabola, or rather, a focal line that runs the length of the trough. The troughs can be tilted from east to west to follow the movement of the sun during the day, maximising exposure.
The fact that the system only needs to tilt on the one axis helps keep costs down. It’s also easy to scale a parabolic system up to produce more power—you can simply add more troughs. A parabolic trough system can produce hundreds of megawatts, and excess heat can be stored in a molten salt reservoir for later use.
The biggest parabolic trough plant in the world is the Solana plant in Arizona, covering an area of around 7.8 square kilometres. The area used for parabolic trough systems must generally be cleared and bulldozed to provide a flat level surface. Currently, around 90 per cent of the world’s CST installations are parabolic trough systems.
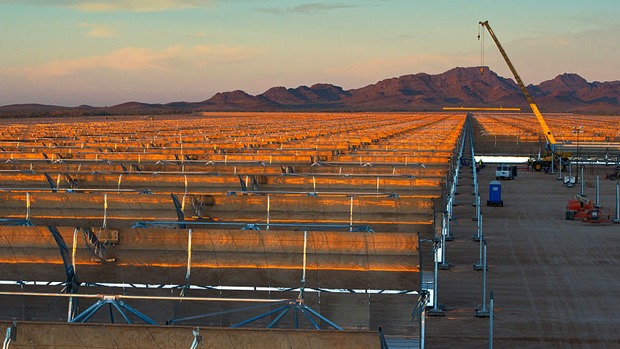
Linear Fresnel reflectors
Linear Fresnel reflectors are another growing solar thermal technology. They are named after the French physicist who described how to approximate the effects of a large lens by simply splitting it up into smaller curved sections laid out on a flat surface. The curvature of the small sections approximately replicates the effect of the entire curved lens surface.
A linear Fresnel reflector system consists of strips of angled mirror laid flat on the ground such that it approximates a parabolic trough system. The resulting array is less efficient but cheaper to build as the mirror sections are supported directly by the ground, rather than needing a large supporting structure. This process uses less material and so reduces construction costs. However, the fact that the effects are only approximately replicated also makes the focal point less sharp, reducing the maximum temperature that can be achieved.
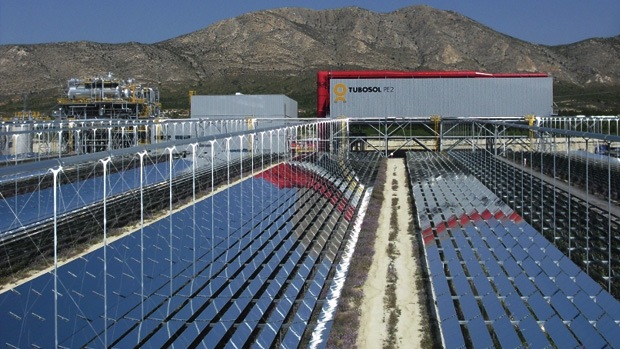
Solar dishes
Solar dishes use a parabolic dish to track the sun and reflect sunlight to a single focal point. The resulting concentration factor can be very high, but the difficulty and cost of building them is also higher than for other systems. They must be constructed so they can track the sun in two directions, tracking both the sun’s elevation that changes throughout the day and over the course of a year. This actually makes them 40 per cent more efficient than other CST approaches.
Although solar dishes are the most efficient means of concentrating solar radiation to produce high temperatures, they have not been widely commercialised. The early development of these systems saw them incorporate a particular type of engine, called a Stirling engine. This made them expensive, and also meant the design could not also include a storage capacity, which has somewhat stymied their further development.
Engineering a system that can support both the dish and its heat receiver and has the capability to track the sun in two directions has limited the size of these systems and they have not been widely commercialised at scale for electricity generation. Pilot projects have so far generally produced less than 100 kW, but there may be a role for solar dishes as efficient, distributed power sources in a future smart grid, or to supplement other energy needs.
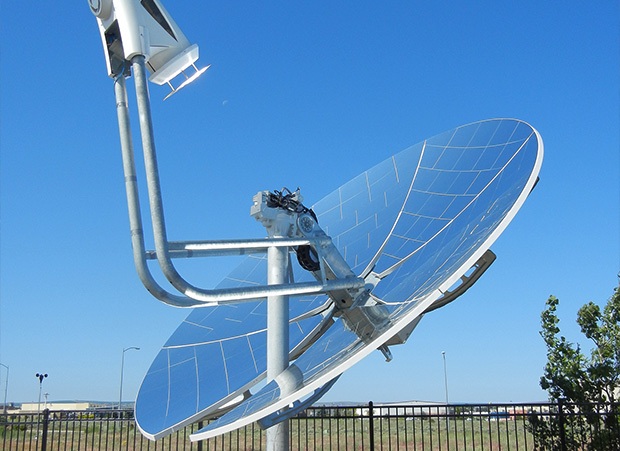
Solar towers
Solar towers are another engineering approach that is rapidly becoming the standard, though they are still behind parabolic troughs in terms of installed capacity. Just as linear Fresnel reflectors are essentially an approximation of parabolic trough systems, the array of mirrors used in solar tower systems are an approximation of a dish shaped system.
Solar towers use vast arrays of mirrors called heliostats (from Greek helios = sun and Latin status = stationary), spread over a large area. The heliostats are stationary in that they are fixed to the ground, but they are built to tilt in two directions so they can track the movement of the sun through the sky, continually reflecting sunlight to the top of a tall tower.
These systems generally concentrate the radiation by around 1,000 times, onto a comparatively small receiver (it’s actually around the size of a house!). Oil can’t withstand the temperatures this can produce so steam and molten salt are used as heat transfer fluids.
So far, commercial tower plants function using temperatures of around 600°C, while experimental systems have reached more than 1,000°C. The practical limit of around 600°C is imposed by the capability of the receiver and heat transfer fluid, rather than the capacity of the heliostat field, or indeed, the amount of sunlight available. Again, excess heat can also be stored for within a molten salt reservoir.
The biggest single solar tower system in the world is the Crescent Dunes plant in Nevada, and its array of 10,347 heliostats covers a total area of around 6.5 square kilometres. The largest complex of solar towers is the Ivanpah system, in the Mojave Desert, California. It has 3 towers and 173,500 heliostats that together cover a total area of around 16 square kilometres.
Solar tower systems have an advantage in that their heliostat arrays can to a large extent work around existing landforms and native vegetation. It’s even possible that they could be placed in grazing lands; their height above the ground means they can provide shade for livestock.
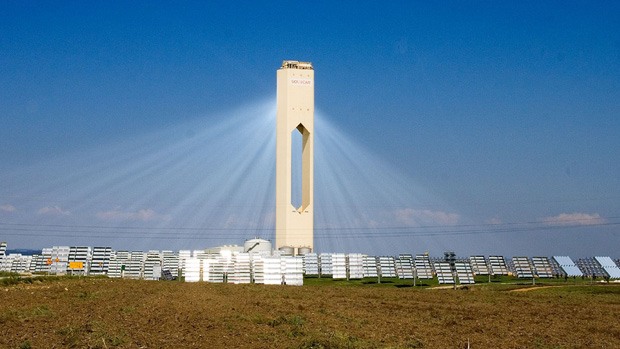
Where to for concentrating solar thermal?
Improving the technology
The future of CST technologies will almost certainly see further development of their use for electricity generation. In terms of improving or optimising the geometry and configuration of the collectors, the technology of the linear systems at least is already at a stage where there’s not much further to go.
At the moment, many more megawatts of heat are collected in CST systems than megawatts of electricity produced. There are two reasons for this: much of the heat is stored for later use, and also because the steam turbines that ultimately convert the heat to electrical energy are only around 40 per cent efficient. So, in terms of electricity generation, the real gains will be made in improving the efficiency of the turbines, perhaps by using new technologies such as air turbines, or carbon dioxide turbines that run at higher temperatures, taking advantage of the high temperatures that CST plants can already produce, yet not practically use.
Other huge gains could be made by the development of advanced materials to act as heat transfer fluids that are able to deal with high temperatures and also stay liquid at ambient temperature. Another development is the use of particles instead of a fluid to transfer the heat. In this system, ceramic particles the size of sand grains and capable of withstanding very high temperatures (and therefore capable of carrying more heat than fluids) are stored at the top of the receiver. They are released and pass through the area of concentrated solar radiation and collected in a storage tank. Thermal energy (heat) is taken from the particles when required, and then the cooler particles are transported back to the top of the receiver by an elevator system.
Not just electricity production
As well as being used to drive industrial chemical processes, CST technologies can also play a role in providing energy for heating or cooling in large buildings, as seen with the facility recently installed on a shopping centre in Ballarat, Victoria.
Another significant use of CST technologies is the production of synthetic fuels, or solar fuels. Solar fuels are liquid or gas compounds that will react with oxygen (typically through combustion) to release energy.
The ‘solar’ part of their name comes from the fact that they are initially produced using solar radiation. They include a range of liquid and gas fuels, such as synthetic diesel, petrol, methanol, ammonia and hydrogen and can be made from either hybrid solar–fossil fuel systems (where solar energy drives the production of syngas from a traditional fossil fuel source) or from completely renewable solar systems (where solar energy drives the production of hydrogen and/or syngas from the dissociation of water and carbon dioxide).
Syngas is used in the same way as traditional natural gas—that is, combusted to release its energy—but it’s a more efficient form of fuel. More about concentrating solar fuels can be found in the CSIRO Solar Fuels Roadmap for Australia.
Upping installed capacity
Although solar thermal is a proven energy technology with a 30-year track record (the first commercial-scale facility was built in California 1984–90, and now has a 354 MW capacity), large-scale facilities have been slow to emerge. A major initiative to encourage renewable energy begun in 2004 by Spain—one of the European countries with the most hours of sunshine—has led to rapid development of the industry.
According to the Renewables 2016 Global Status Report, by the end of 2015 global installed capacity stood at 4.8 GW with Spain (2,300 MW) and the United States (1,738 MW) solid leaders.
Africa is currently a hotspot (pardon the pun!) of new CST activity, with Morocco at the forefront of new capacity. The Noor I plant, capable of producing 160 MW, came online in 2015. This is part of the Noor-Ouarzazate complex, which when fully online in 2018 will produce 500 MW. There are ideas to harness solar power from sun-rich North Africa and the Middle East, and transfer the electricity via high-voltage transmission to consumption centres in Europe. These advances in Morocco are the first steps, and the country is already connected to Spain by an undersea cable. 200 MW of capacity was also realised in South Africa, with the 100 MW KaXu Solar One, the 50 MW Bokpoort and the 50 MW Khi Solar One plants all coming online in 2015 and early 2016. The United States also added another 110 MW, with the Crescent Dunes solar tower installation.
China has also announced a plan to add 1 GW of CST power within the coming years.
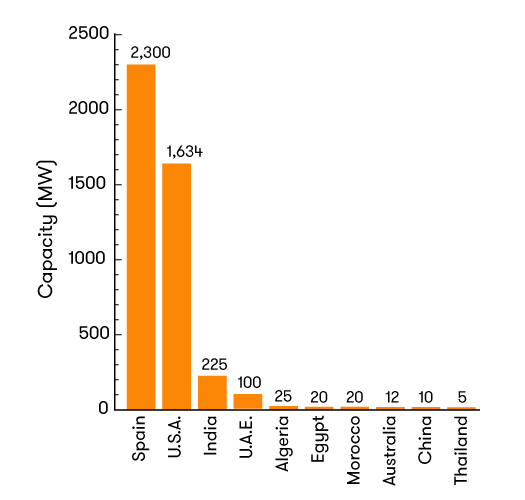
Global concentrating solar thermal power capacities, 2015.
Source: REN21 Renewables 2016 Global Status Report (table R7).
CST electricity production at present accounts for only a small share of the world’s renewable energy production. However, it’s interesting to note that the growth of CST is following a very similar trajectory to those of both wind power and solar pv. Each of these technologies started off on very small scales, then slowly gained momentum as the science advanced and costs came down.
Although at first blush CST appears to be more expensive than other renewable technologies, the added bonus of the storage that that can be so easily built into a CST plant must also be taken into account. It gives CST plants the sort of versatility that will be needed if we truly wish to power our future with renewable technologies. CST probably won’t be able to do it on its own, but it certainly offers a valuable contribution to the mix.